2.2.1 Systematic position
a. Taxonomy
Cronquist (1988), Thorne (1992) and Takhtajan (1997) suggest
following water hyacinth taxonomic placement (Center et al. 2002):
Division: Magnoliophyta
Class: Liliopsida
Subclass: Commeinidae
Superorder: Commelinanae
Order: Pontederiales
Family: Pontederiaceae
Genus: Eichhornia
Specific epithet: crassipes (Martius) Solms-Laubach.
b. Morphology
Water hyacinth (Eichhornia crassipes) is a perennial,
floating macrophyte, freshwater aquatic vascular plant with rounded, upright,
shiny green leaves and spikes of lavender flowers (Reed et al., 1997).
The petioles of the plant are spongy with many air spaces and contribute to the
buoyancy of the hyacinth plant. When grown in wastewater, individual plants
range from 0.5 to 1.2 m from the top of the flower to the root tips (Reed
et al., 1997).
The plants spread laterally until the water surface is covered
and then the vertical growth increases. Hyacinths are very productive
photosynthetic plants. Their rapid
growth is a serious nuisance problem in many slow flowing
southern waterways. These same attributes become an advantage when used in a
wastewater treatment system.
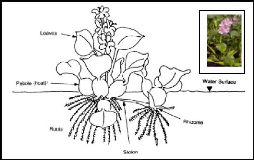
Figure 2.2: Morphology of water hyacinth plant (source:
Aquatics, 2005)
2.2.2 Ecological factors
Water hyacinth is heliophyte plant growing best in warm waters
rich in macronutrients. Optimal water pH for growth of this aquatic plant is
neutral but it can tolerate pH values from 4 to 10. This is very important fact
because it points that E. crassipens can be used for treatment of
different types of wastewater. Optimal water temperature for growth is
28-30oC. Temperatures above 33oC inhibit further growth
(Center et al., 2002). Optimal air temperature is 21-30oC.
So if aquatic systems with water hyacinth are constructed in colder climates it
would be necessary to build greenhouses for maintaining optimal temperature for
plant growth and development. Low air humidity from 15% to 40% can also be
limiting factor for undisturbed growth of water hyacinth (Allen, 1997). E.
crassipens tolerates drought well because it can survive in moist
sediments up to several months (Center et al., 2002).
2.2.3 Potentials and constraints in using of water
hyacinth
Water hyacinth is plant with many advantages firstly because it
can be used for many purposes, but it has one major consequence. E.
crassipes is one of the most invasive weeds that can destroy precious
aquatic ecosystems in a short time which can lead to
series of other problems. Because of that it is very difficult
to answer the question - Is E. crassipes the golden plant or the
world's worst aquatic weed?
One can often read that people have a moral imperative to
think about potential utilization of abundantly available biomass of water
hyacinth in tropical countries for the benefit of people for whom E.
crassipes has created many problems or even has destroyed their lives.
There are many examples around the world of how communities or individuals have
used water hyacinth to great advantage. In regions where it can be found in
abundance water hyacinth can be used like food for people because its leaves
are rich in proteins and vitamin A. It can be also utilized as green fertilizer
or as mulch, compost and ash in regenerating degraded soils. (Lindsey and Hirt,
1999).
In African countries like Uganda water hyacinth has also
influenced on much frequent occurrence of diseases (dysentery, malaria, and
schistosomiasis) related to content of different pathogens in water. It has
been discovered that water hyacinth's quest for nutrients can be turned in a
more useful direction. The plant has been shown to accumulate trace elements
such as Ag, Pb, Cd and Zn.
The focus on water hyacinth as a key step in wastewater
recycling is due to the fact that it forms the central unit of a recycling
engine driven by photosynthesis and therefore the process is sustainable,
energy efficient and cost efficient under a wide variety of rural and urban
conditions.
2.3 Heavy metals
The designation «Heavy metals» is applied to a group
of metals and metalloids with a specific density greater than 5 g /
cm3. They are frequently associated with pollution and toxicity in
the environment. In general «trace metals» is the term used because
they occur in low concentrations in the earth's crust. Element like As, Cd,
CrVI, Hg and Pb are known to be very toxic. However some metals like
Co, Cu, Mn, Se and Zn are essential for living organisms at low concentrations
and are vital components of enzymes. Heavy metals occur naturally in the
environment, usually at relatively low concentrations as a result of weathering
and other pedogenic processes acting on the
rock fragments on which soils develop (Rulkens et
al., 1995). These metals are then transported to the aquatic ecosystem
through leaching and run-off phenomena.
Human activities have increased the amount of heavy metals
released to the environment. Metals have been exploited at an alarming rate
because of their economic value. The negative consequences of this situation
have only been realized within the last decades. Because of that, many
researches on the interaction of heavy metals with various components of the
environment have been conducted and still going on with the main objective of
finding suitable ways of solving and avoiding heavy metals pollution on the
surrounding environment.
The existence of heavy metals in the environment represents a
very significant and long-term environmental hazard. Even at low concentrations
these metals can be toxic to organisms, including humans. In particular,
chromium is a contaminant that is a known mutagen, teratogen and carcinogen
(Chang, 1996; Young et al., 2006).
The removal of heavy metals from aqueous solutions has
therefore received considerable attention in recent years. However, the
practical application of physicochemical technology such as chemical
precipitation, membrane filtration and ion exchange is sometimes restricted due
to technical or economical constraints. For example, the ion exchange process
is very effective but requires expensive adsorbent materials (Lehmann et
al., 1999; Volesky, 2001).
The use of low-cost waste materials as adsorbents of dissolved
metal ions provides economic solutions to this global problem and can be
considered an eco-friendly complementary (Volesky et al., 1995; Mullen
et al., 1989). At present, emphasis is given to the utilization of
biological adsorbents for the removal and recovery of heavy metal contaminants.
(Young et al., 2006).
Aquatic macrophytes are known to remove metals by surface
adsorption and/or absorption and incorporate them into their own system or
store them in a bound form (Rai et al., 1995). The uptake of trace
metals by the root systems of aquatic plants depend both on the kind of metal
and on the species of plant absorbing the metal (Samecka-Cymermann and Kempers,
1996).
|