CHAPITRE VI ETUDE SPECIFIQUE SUR LE DEVENIR DE DEUX
DESINFECTANTS LARGEMENT UTILISES DANS LES HOPITAUX : L'HYPOCHLORITE DE SODIUM
ET LE GLUTARALDEHYDE
I. Introduction
Les résultats des bioessais pratiqués sur les
effluents hospitaliers, montrent ont l'écotoxicité d
élevée de ces effluents sur les organismes aquatiques
(GARTISER et ai, 1996; LEPRAT, 1998;
JEHANNIN, 1999). Par ailleurs, la mise en oeuvre des tests de
mutation génique AMES et HAMSTER prouve que les effluents des services
cliniques et des laboratoires hospitaliers peuvent présenter un
caractère de mutation génique (GARTISER et ai, 1996).
L'une des hypothèse avancée sur la toxicité des rejets
liquides hospitaliers est qu'elle est due aux désinfectants
utilisés dans les services (DELOFFREBONNAMOUR, 1995;
JouBois et al., 2002).
Parmi les différents désinfectants, les produits
à base de glutaraldéhyde et les désinfectants à
base de chlore (eau de Javel) sont largement utilisés dans presque tous
les hôpitaux du monde. Il a été donc décidé,
dans le cadre de cette thèse de réaliser une étude
spécifique sur le devenir de l'hypochlorite de sodium et du
glutaraldéhyde contenus dans les effluents hospitaliers. L'objectif de
ce chapitre est de présenter les résultats de l'étude
spécifique.
Ce chapitre est divisé en deux articles de:
1- Evens Emmanuel, Yves Perrodin, Gérard Keck,
Jean-Marie Blanchard, Paul Vermande. Toxicological Effects of Sodium
Hypochlorite disinfections and its contribution to AOX formation in hospital
wastewater. 2003, (accepted Envfronment International)
2- Evens Emmanuel, Khalil Hanna, Christine Bazin,
Gérard Keck,Yves Perrodin. Single and combined effects of glutaraldehyde
with surfactants on aquatic organisms: termodynical and toxicological
approaches to predict fate of pollutant mixtures on environment.
Chapitre VI Etude spécifique sur le devenir de
deux désinfectants largement utilisés dans les hôpitaux :
l'hypochlorite de sodium et le glutaraldehyde
II. Toxicological effects of sodium hypochiorite
disinfections on aquatic organisms and its contribution to AOX formation in
hospital wastewater
Evens EMMANUEL1r2*, Yves
PERRODIN1, Gérard KECK3, Jean-Marie
BLANCHARD2, Paul VERMANDE2
1 Laboratoire des Sciences de l'Environnement,
École Nationale des Travaux Publics de l'État, Rue Maurice Audin,
69518 Vaulx-en-Velin, France
2 Laboratoire d'Analyse Environnementale des
Procédés et Systèmes Industriels, Institut National des
Sciences Appliquées de Lyon, 20 avenue Albert Einstein, 69621
Villeurbanne Cédex, France
3 Unité d'Ecotoxicologie, Ecole Nationale
Vétérinaire de Lyon, BP 83, 69280 Marcy l'Etoile, France
Keywords : Sodium hypoclorite, AOX,
hospital effluents, toxicity, Daphnia.
* Corresponding author. Tel : +(33) 4 72 04 72 89; fax:+(33) 4 72
04 77 43 E-mail address : evemml eyahoo.fr
Chapitre VI Etude spécifique sur le devenir de
deux désinfectants largement utilisés dans les hôpitaux :
l'hypochlorite de sodium et le glutaraldehyde
II.1. Abstract
Sodium hypochlorite (NaOCI) is often used for disinfecting
hospital wastewater in order to prevent the spread of pathogenic
microorganisms, causal agents of nosocomial infectious diseases. Chlorine
disinfectants in wastewater, react with organic matters to give rise to organic
chlorine compounds such as AOX (halogenated organic compounds adsorbable on
activated carbon), which are toxic for aquatic organisms and persistent
environmental contaminants. The aim of this study was to evaluate the toxicity
on aquatic organisms of hospital wastewater from services using NaOCI in
prechlorination. Wastewater samples from the infectious and tropical diseases
department of a hospital of a big city of the southeast of France were
collected. Three samples per day were done in the connecting well department at
9 A.M, 1 P.M. and 5 P.M. during eight days
from 13 March to 22 March 2001, and a mixture was made at 6 P.M.
with the three samples in order to obtain a representative sample for
the day. For toxicity test, the 24-h EC50 on Daphnia magna
and a bioluminescence assay using Vibrio fischeri photobacteria
were used. Fecal coliforms and physicochemical analysis such as: Total Organic
Carbon (TOC), chloride, AOX, Total Suspended Solids (TSS) and Chemical Oxygen
Demand (COD) were carried out. Wastewater samples highlighted an important
acute toxicity on Daphnia magna and Vibrio
fischeriphotobacteria. However, low most probable number (MPN) ranging
from <3 to 2400 for 100 mL were detected for fecal coliforms. Statistical
analysis, at a confidence interval of 95%, gave a strong linear regression
assessed with r= 0,98 between AOX concentrations and EC50 (TU) on
daphnia. The identification of an ideal concentration of NaOCI in disinfecting
hospital wastewater, i.e. its NOEC (Non Observed Effect Concentration) on algae
and Daphnia magna, seems to be a research issue which could facilitate
the control of AOX toxicity effects on aquatic organisms. Therefore, it would
be necessary to follow-up at various dosages the biocide properties of NaOCI on
fecal coliforms and its toxicity effects on aquatic organisms.
11.2. Introduction
Sodium hypochlorite (NaOCI, CAS no. 7681-52-9 and EC no.
017-011-00-1), a solution containing between 12.5 to 25% active chlorine gas
(Cl2), has a wide range of domestic, industrial, scientific and biomedical
applications related to biocide properties (U.S. EPA, 1994a; Brondeau et al.,
2000). When NaOCI is added to water and wastewater, the solution reacts readily
with biological materials (including proteins and nucleotide bases) to produce
a variety of organic chlorinated compounds (U.S. EPA, 1989a), which are mostly
lipophilic, persistent, and toxic in aquatic environment (SalkinojaSalonen and
Jokela, 1991).
The medical use of chlorine began in 1826 with chlorine water
in delivery wards to prevent puerperal fever, and chloroform was first used for
medical anesthesia in 1847 (Carey et al, 1998). Nowadays, the
widespread biomedical use of NaOCI is particularly for the local surface and
toilet disinfections, based on it very broad spectrum of biocide activity
against bacteria, virus and fungi (DeloffreBonnamour, 1995).
Chapitre VI Etude spécifique sur le devenir de
deux désinfectants largement utilisés dans les hôpitaux :
l'hypochlorite de sodium et le glutaraldehyde
In hospital, besides sodium hypochlorite, a variety of
substances such as pharmaceuticals, radionuclides, solvents, are in use for
medical purposes as diagnostics, disinfections and research (Erlandsson and
Masson, 1978; Richardson and Bowron, 1985; Kümmerer et al, 1997).
After application, many non-metabolized drugs are excreted by the patients and
enter into wastewater (Halling-Sorensen et al, 1998). After their use
disinfectants also reach the wastewater (Kümmerer, 2001). Due to
laboratory and research activities or medicine excretion into wastewater,
hospitals may represent an incontestable release source of many toxic
substances in the aquatic environment (Jolibois et al, 2002).
Hospital effluents reveal the presence of organochlorine
compounds in high concentrations (Leprat, 1998). AOX up to 10 mg/L were proved
in the effluents of the hospitalization services of a university hospital
center (Gartiser et al, 1996). Presence of AOX (adsorbable organic
halogens) in hospital wastewater is usually associated with the presence of
iodinated X-ray contrast media release by hospital radiography department. The
assessment of AOX shows that those non conventional pollutants have a bad
biodegradability and a bad behavior of adsorption (Sprehe et al,
1999).
Throughout the world, the assessment of wastewater discharges
or effluents is focused on the precautionary principle, i.e., reduction of
specific pollutants or substances in the framework of their emission policies
(Kinnersley, 1990). In Directive 98/15/EEC, the European Commission (1998)
proposes an emission limit of pollutants in wastewater for all member states.
Based on a comparison of the measured values for chemical parameters in
effluents and the proposed limited values, the directives on wastewater
management in their application most often do not allow to evaluate the
toxicity effects of hazardous substances, contained particularly in hospital
wastewater, on organization levels of the aquatic food chains (Perrodin, 1988).
However, a quick assessment of toxic effects of substances on aquatic organisms
is possible by completing chemical characterization with toxicity assay
(Perrodin, 1988, Schowanek et al, 2001, Sponza, 2003).
Cladocerans, a group of freshwater zooplankton, are broadly
distributed in freshwater bodies, are present throughout a wide range of
habitats, and are important links in many food chains (Abe et al.,
2001). Daphnia sp. are extensively used to investigate the acute
and chronic toxicity of industrial and agricultural chemicals in aquatic
ecosystems (OECD, 1984; ASTM, 1994; Verschueren, 1996; Hanazato, 1998). Because
they have a relatively short life cycle, require little space, are adaptable to
laboratory conditions and are sensitive to a broad range of aquatic
contaminants (Cooney, 1995). Application of Daphnia magna bioassay procedures
on hospital wastewater had proved the high toxicity of these wastewater
(Jehannin, 1999; Emmanuel et al, 2001). One of the assumptions on this
toxicity is the presence of chlorinated disinfectants. The application of
chlorine as a general oxidant in sewage treatment industries results in the
creation of mixtures, AOX formed during chlorine disinfections of humic-rich
raw water (Jokela et al., 1992), that are also emitted in the environment
(Carey et al., 1998). The aim of this study was (i) to make a brief review of
the chemistry and the
Chapitre VI Etude spécifique sur le devenir de
deux désinfectants largement utilisés dans les hôpitaux :
l'hypochlorite de sodium et le glutaraldehyde
toxicology of chlorinated disinfectants in order (ii) to evaluate
the toxicity on aquatic organism of hospital wastewater from services using
NaOCI disinfectant.
11.3. The chemistry and toxicology of chlorinated
disinfectants in water and wastewater 11.3.1. Chemical behavior of chlorinated
disinfectants
In spite of the concern about the formation of toxic
by-products usually associated with it use (Marhaba and Washington, 1998; Lopez
et al, 2001), Chlorine (Cl2) and its derive NaOCI are still
the most widely used disinfectant (Baxter, 1994). When chlorine gas is
dissolved in water, it hydrolyses rapidly according to the following equation
to yield hypochlorous acid:
Cl2 + H2O --> HCIO + H+ + CI-
(1)
Hypochlorous acid is also formed when sodium hypochlorite (NaOCI)
is used as the source of chlorine: NaOCI + H2O --> HCIO +
Na+ + OH- (2)
Hypochlorous acid is a weak acid, and will undergo partial
dissociation as follows:
HCIO --> H+ + CIO- (3)
The ratio [HCIO]/[ C10-] is pH dependent (Lopez et
al, 2001). In fact, in presence of pH between 6 and 9, both
hypochlorous acid and hypochlorite ion will be present in waters; the
proportion of each species is depending also on temperature (White, 1986).
Hypochlorous acid is significantly more effective as a biocide than the
hypochlorite ion. If bromide ions are present, chlorine will also oxidise
bromide to form hypobromous acid (Von Guntent and Hoigné, 1995):
HCIO + Br <=> HBrO + Cl-- (4)
Hypobromous acid is an effective biocide, in this context the
group [HBrO]/[Br0-] are better oxidants than [HCIO]/[CI01,
conversely, hypochlorous acid and hypochlorite ion are better halogenating
substances (Lopez et al, 2001). When ammonia is also present, the
competing reactions of chlorine with bromide and ammonia are likely to result
in the rapid formation of both monochloramine and hypobromous acid. A number of
other reactions can then occur:
NH2CI + Br + 2H2o -> HOBr +
NH4OH + Cl- (5)
HOBr + NH4OH --> NH2Br + 2H20
(6)
NH2Br + HOBr --> NHBr2 + H2O
(7)
HCIO and CIO- can react with organic compounds by
addition, substitution and oxidation (Boyce and Hornig, 1983). The reaction of
chlorine with organic constituents in aqueous solution can be grouped into
several types:
(a) Oxidation, where chlorine is reduced to chloride ion,
e.g.
RCHO + HCIO --> RCOOH + H+ + Cl- (8)
(b) Addition, to unsaturated double bonds, e.g.
RC=CR' + HCIO --> RCOHCCIR' (9)
(c) Substitution to form N-chlorinated compounds, e.g.
RNH2 + HCIO --> RNHCI + H2O (10)
or C-chlorinated compounds, e.g.
Chapitre VI Etude spécifique sur le devenir de
deux désinfectants largement utilisés dans les hôpitaux :
l'hypochlorite de sodium et le glutaraldehyde
RCOCH3 + 3HCIO --> RCOOH + CHCI3
+ 2H20 (11)
Chlorine substitution can lead to the formation of
halogenated compounds, such as chloroform (e.g. equation 11). Although such
reactions are significant in terms of the resultant halogenated byproduct, it
has been estimated that only a few percent of the applied chlorine ends up as
halogenated organic products (White, 1986).
11.3.2. Environmental fate and toxicological effects of
chlorinated disinfectants
In addition to the chlorinated water that is released
into the environment from sewage treatment operations and other sources,
chlorine is potentially released during its transport (NTP, 1992). Limited
monitoring studies have detected chlorine in the ambient atmosphere at
concentrations ranging from 1 to 3.7 mg/m3 or 0.344 to 1.27 ppm
(U.S. EPA, 1994a). Chlorine may react with soit components to form chlorides;
depending on their water solubility, theses chlorides are easily washed out
from the soit (Seiler et ai, 1988). U.S. EPA (1989a) reports that
chlorine hydrolyses very rapidly in water (rate constants range from 1.5x10-4
at 0°C to 4.0x10-4 at 25°C). However, there is no potential for the
bioaccumulation or bioconcentration of chlorine (U.S. EPA, 1994a).
Chlorine gas (Cl2) or sodium hypochlorite (NaOCI)
added to drinking water effectively inactivates bacteria in 20 minutes at
concentrations of 0.03 to 0.06 mg/L at pH range of 7.0 to 8.5 and temperature
range of 4°C to 22°C (NTP, 1992). Drinking water concentrations of
higher than 90 ppm chlorine caused irritation of human membranes of throat and
mouth (U.S. EPA, 1989a). In fact, chlorine is a primary irritant to the mucous
membranes of the eyes, nose and throat and to the linings of the entire
respiratory tract (Stokinger, 1982). Mice and rats exposed to chlorine at the
RD50 concentration (9-11 ppm, 6h/day for 1, 3, or 5 days) developed
degeneration of olfactory sensory cells in the olfactory mucosa, loss of cilia
of the respiratory epitlelium, and cellular exfoliation primarily of the naso
and maxilloturbinates (U.S. EPA, 1994a).
The major target organs for the subchronic/chronic
toxicity of chlorine in humans are the respiratory tract and the blood. In
animais, the major target organs are the immune system, the blood, the
cardiovascular system and the respiratory tract (U.S. EPA, 1994a). EPA has
derived an oral RfD (reference dose) of 0.1 mg/kg/day for chlorine, based on a
no-obeserved-effect level of 14.4 mg/kg/day in a chronic drinking water study
in rats (U.S. EPA, 1994b).
No conclusion on the carcinogenicity and on the
developmental/reproductive toxicity of chlorine can be made on the limited
information available from human and animal studies (NTP, 1992; U.S. EPA 1989
and 1994b). However, chlorine was mutagenic in Salmonella typhimurium strains,
without metabolic activation, produced chromosome aberrations in human
lymphocytes and other mammalian cells; interacted with DNA in E. coli polA (as
sodium hypochlorite) and was negative for the induction of erythrocyte
micronuclei or chromosome aberrations of bone narrow cells mice (up to 8
mg/kg/day of NaOCI) for up to 5 days (U.S. EPA, 1989a).
Chapitre VI Etude spécifique sur le devenir de
deux désinfectants largement utilisés dans les hôpitaux :
l'hypochlorite de sodium et le glutaraldehyde
Chlorine has high acute toxicity to aquatic organisms. Many
toxicity values are less than or equal to 1 mg/L. Table 1 shows obtained
results on toxicity studies of chlorine on some aquatic species (AQUIRE, 1994).
Papillomas of the oral cavity in fish have been associated with exposure to
chlorinated water supplies (NTP, 1992). Low level chlorination (0.05 to 0.15
mg/L) results in significant shifts in the species composition of marine
phytoplankton communities (U.S. EPA, 1994a).
Chlorine is phytotoxic but is also essential to plant growth;
crops need around 5 pounds or more of chlorine per acre (U.S. EPA, 1994a).
Acute toxicity to plants is characterized by defoliation with no leaf symptoms
and, in higher plants forms, by spotting of the leaves at 1.5 mg/m3,
and marginal and interveinal injury at 150-300 mg/m3 (Seiler et al.,
1988).
Table 1. Toxicity of chlorine on aquatic organisms
(AQUIRE, 1994)
Species Duration of tests Range of EC50 or
LC50
Daphnia magna (cladocerans) 24-hour 0.076 -- 0.16
mg/L
Daphnia pu/ex(cladocerans) 24-hour 0.005 -- 0.1 mg/L
IVitrocra spinipes (snail) 48-hour 5.3 -- 12.8 mg/L
Oncorhynchus mykiss(rainbow trout) 96-hour 0.13 -- 0.29
mg/L
Salvelinus fontinalls (brook trout) 96-hour 0.1 -- 0.18
mg/L
Lepomis cyan//us (green sunfish) 96-hour 0.71 -- 0.82
mg/L
11.4. Materials and methods
11.4.1. Sampling and pH measurements
The wastewater from the infectious and tropical diseases
department (ITDD), with a capacity of 144 beds, of a hospital of a big city of
the southeast of France have been selected. Wastewater was collected before
entering into the entire hospital sewer network, which discharges the total
volume of effluents from the various departments into the urban wastewater
network without pre-treatment. This choice was justified by the fact that the
ITDD collector does not receive effluents containing iodized X-ray contrant
media from radiography department, substances which could be contributed to AOX
concentrations. Three samples per day were done in the connecting well
department at 9 A.M, 1 P.M. and 5 P.M. during
eight days from 13 March to 22 March 2001. A mixture has been made with the
three samples at 6 P.M. in order to obtain a representative
sample for the day. In the objective to avoid or decrease volatility process in
samples, laboratory conditions controlling flow and turbulence have been
considered during mixture preparations. Water sample was collected by means of
a telescopic perch in a 1-L glass flask. pH was measured directly on site after
sampling with a pH meter HANNA instrument HI 8417 (accuracy pH #177; 0.01pH, mV
#177; 0.2 mV #177; 1, °C #177; 0.4°C) digit and standard electrode HI
1131 B (refillable glass combination pH electrode). All the water samples and
the mixture were kept at 4°C until analysis.
Chapitre VI Etude spécifique sur le devenir de
deux désinfectants largement utilisés dans les hôpitaux :
l'hypochlorite de sodium et le glutaraldehyde
11.4.2. Chemical analysis
Total suspended solids (TSS) concentrations were
determined in conformity with the European standard NF EN 872 (AFNOR, 1997a)
after filtration through a 1.2 pm membrane and dewatering at 105
°C.
Chlorides were determined by following the European
standard NF EN ISO 10304-1 (AFNOR, 1997b) on diluted and filtered samples at
0.45 pm by using DIONEX DX-100 ion chromatograph with suppressed conductivity
detection from 0.0 to 1000 pS. Ionpac AS14 4x250 mm analytical column (P/N
046124) was used for chloride sample analysis. AOX were measured according to
European standard EN 1485 (AFNOR, 1997a). COD was measured by potassium
dichromate method using HACH spectrophotometer 2010 and test procedure provided
by the supplier. Because hospital wastewater may record temporal
physicochemical evolutions, measures of COD on the three samples and the
mixture COD were determined for wastewater samples of March 20, 2001. This
decision has been taken in order to analyze the variations of COD during the
day.
Total Organic Carbon (TOC) was carried out on filtered
samples at 0.45 pm and pre-treated with orthophosphoric acid
(H3PO4). French standard T90-102 (AFNOR, 1997a) was followed by
using a carbon analyzer SPECTRA France, LABTOC model, with potassium per
sulfate reagent (K2S208) and UV oxidation.
11.4.3. Toxicity test procedures
The determination of the inhibition of Daphnia
magna mobility is a acute toxicity assay. Its objective is to identify the
initial concentration of a pollutant in solution or an aqueous mixture which
may immobilize in 24 or 48 hours 50% of exposed daphnia into polluted
solutions. According to the European standard NF EN ISO 6341 (AFNOR, 1997c),
the different assays were carried out on Daphnia sp. maintained in
parthenogenetic culture in the laboratory (POLDEN of the National Institute of
Applied Sciences of Lyon -- INSA de Lyon). The sensibility of the laboratory
species was controlled by regular tests with potassium dichromate. Young female
Daphnia, aged less 24 h were only used. The normal medium, without EDTA, was
also used. The essays were realized at 20 #177; 2°C under darkness
condition. All the assays were carried out in a limit of time from 6 to 48 h
after sampling.
Because hospital wastewater is considered as toxic for
aquatic environment, a volume of 250 mL unfiltered samples was taken for each
assay. In order to understand the effects of color, turbidity and TSS present
in hospital effluent samples on Daphnia sp., the toxicity of 250 mL
filtered sample (0.45 pm pore size membrane) was studied by comparing the
results with the unfiltered volume of the same sample. The three required
conditions for the validity of assays were observed: (i) the concentration of
dissolved oxygen (DO), in the control group, was 2 mg/L at the end of each
assay; (ii) the
Chapitre VI Etude spécifique sur le devenir de
deux désinfectants largement utilisés dans les hôpitaux :
l'hypochlorite de sodium et le glutaraldehyde
observed percentage of immobilization in the control group
vessels was 10%; (iii) EC50 in 24h of potassium dichromate was
between 0.6 to 1.7 mg/L.
The bioassay on bacteria luminescence MICROTOX was carried out
with a LUMIStox system (Dr Lange GmbH, Duesseldorf, Germany) following the
standard procedure of the European standard NF EN ISO 11348-3 (AFNOR, 1999).
Tests were performed using gram negative marine bioluminescent bacteria of the
species Vibrio fischeri NRRL-B-11177 of the Vibrionaceae
family. In order to prevent the interferences of TSS on the bacteria
luminescence, samples were filtered using a 0.45pm pore size membrane. The
samples were treated with NaCI solution of 20 g/L and brought to 50 mS/cm of
conductivity before the analysis. Starting from the concentration of the
sample, eight consecutive elutions were tested (dilution factor 1:2); the
inhibition of bioluminescence was measured at a wavelength of 490 nm, with
readings after 5 and 15 minutes of incubation at 15 °C. The
EC50 values were calculated as reported by Bulich (1979).
11.4.4. Statistical data analysis
The immobilization percentage, of the initial total number of
20 daphnia used in the test, were determined for each sample
concentration. EC50 values for inhibition assays by
Litchfield-Wilcoxon statistic method or probit analysis (Finney, 1971). Results
of CE50 24h, with their confidence interval, are expressed in
percentage of sample dilution in toxic unit TU (1 TU = 100 /
CE50) ·
Multiple regression analysis between y and x
variables was performed using the statistical package Xlstat version 5.0
(b8.3) for Windows (Addinsoft, 2001). The multiple regression STEPWISE
(Agresti, 1990) was used to determine the correlations between AOX (4 and
EC50 24h on daphnia (y). The linear regression was assessed with r,
r2 and adjusted coefficient of determination. r, r2 are
respectively the coefficient of correlation and the coefficient of
determination. Ail the statistical analysis were at a confidence interval of
95%.
11.5. Results
In all samples (simple and mixture) pH was always in an
alkaline range (7.9 -- 8.8) with a variation lower than 1 pH unit. The hospital
wastewater composition from the infectious and tropical diseases department
refers to the amounts of its physical, chemical constituents. These elements
have been determined by measuring COD, TOC, TSS, pH, chlorides, AOX coupled
with the bioassays of toxicity. The European Commission Directive 98/15/EC
(1998) proposes medium discharge standards for all these parameters. The
results of physicochemical characterization of hospital wastewater mixture
samples are summarized in Table 2.
11.5.1. Physicochemical characterization of ITDD
wastewater
In the effluent samples, COD concentrations ranged from 362 to
1492 mg/L exceeded the discharge standards. This could be attributed to the
presence of toxic substances. The COD threshold values for
Chapitre VI Etude spécifique sur le devenir de
deux désinfectants largement utilisés dans les hôpitaux :
l'hypochlorite de sodium et le glutaraldehyde
industrial wastewater which must be reached the sewer network
is given by the Directive 98/15/EC and accounts 125 mg/L (European Commission,
1998). COD concentrations for punctual samples were compared with COD
concentration of the mixture sample. The mixture sample value was different of
mean value of the punctual samples. The variation of COD for this specific
sample day is presented in figure 1.
Table 2. Physicochemical characterization of mixture
samples of HWW
Parameters Units
pH U
Chlorides mg/L
AOX mg/L
TSS mg/L
COD mg/L
TOC mg/L
COD/TOC
Means
|
Minima
|
Maxima
|
SD
|
n
|
8.4
|
7.9
|
8.8
|
0.3
|
8
|
185
|
63.4
|
359.2
|
90.4
|
8
|
0.7
|
0.38
|
1.24
|
0.28
|
8
|
225
|
155
|
298
|
64
|
5
|
638
|
362
|
1492
|
435
|
6
|
218
|
160
|
350
|
78
|
6
|
3.43
|
2.01
|
4.26
|
0.92
|
6
|
1600
|
|
|
|
1400
|
|
1492
|
|
|
|
|
|
|
|
1417
|
1200
|
|
|
|
|
1000
|
|
|
|
|
|
|
|
15)
|
|
1029
|
|
|
|
|
800
|
|
|
|
|
|
|
|
|
792
|
0
|
|
|
|
|
|
|
0
|
|
|
|
|
|
|
|
|
0 600
|
|
|
|
|
|
|
|
|
400
|
|
|
|
|
|
|
|
|
200
|
|
|
|
|
|
|
|
|
0
|
|
|
|
|
m
|
|
a
|
|
9:00 A.M. 1:00 P.M. 5:00 P.M. Mixture - 6:00
P.M.
Collecting time of samples
Figure 1: Variations of COD concentrations between the
three samples and mixture of 6th day
In conventional urban wastewater, TOC concentrations usually
ranged from 80 to 290 mg/L (Metcalf and Eddy, 1991). In the hospital
wastewater, TOC concentrations were from 160 to 350. The difference could be
due to higher chloride concentrations in hospital wastewater. Seiss et al.
(2001) reported that TOC degradation rate depends on the chloride concentration
of the wastewater. The
Chapitre VI Etude spécifique sur le devenir de
deux désinfectants largement utilisés dans les hôpitaux :
l'hypochlorite de sodium et le glutaraldehyde
different organochlorine compounds resulting from NaOCI
disinfections of hospital wastewater could contribute to high TOC
concentrations in these effluents.
TSS concentrations ranged from 155 to 298 mg/L and chloride
values from 63.4 to 359.2 were detected. The linear regression analysis between
TSS (y dependent variable) and chlorides (x independent variable) in the
effluents revealed an acceptable linear correlation (r = 0.93, r2 =
0.86, Durbin-Watson statistic DW = 1.23, P = 0.02). Chlorides and TSS levels
were higher in the effluents samples where higher acute toxicity on Daphnia
magna was observed. The same result was observed for AOX which
concentrations ranged from 0.38 to 1.24 mg/L. The AOX permissible level 1 mg/L,
given in French wastewater regulations (MATE, 1998), is exceeded in higher AOX
concentration in the effluent mixture sample. However, AOX results generated by
chlorine disinfections of hospital wastewater were lower than AOX concentration
of 10 mg/L determined (Sprehe et al., 1999) in hospital wastewater containing
iodized contrast media.
11.5.2. M icrobiological and toxicological
characterizations of ITD D wastewater
Low concentrations of bacteria fora 2.4x103/100mL
were deducted for the hospital effluents (Table 3). Previous studies on
microbiological characterization of hospital wastewater (Bernet and Fines,
2000) reported these effluents have bacteria concentrations lower than
108/100mL generally present in the municipal sewage system (Metcalf
and Eddy, 1991).
MICROTOX results in toxic unit (TU) explain the toxicity of
hospital wastewater on V. fischery. Significant differences were
observed between 5 and 15 min EC50 values, showing that 5 min assay
can be considered as no toxic because all the results are lower than 2 TU. The
results of 15 min ranged from 2.47 to 4.15 TU greater than 2 TU had a toxicity
similar to domestic wastewater (Table 3). However, all the results were lower
than the means of 6.75 TU reported by the literature for the toxicity of
hospital wastewater on V. fischerycompleted after 30 min of exposure
(Jehannin, 1999).
The 24-h EC50 values determined for each mixture
sample toxicity on Daphnia magna, with the correspondent 95%
confidence limits. The results are also presented in Table 3. In all the
samples, the 24-h EC50 values on Daphnia magna ranged from
9.8 to 116.8 TU greater than that 2 TU value proposed by French water agencies
for industrial wastewater discharge. Potential toxicity is a suitable
description of these effluents.
Chapitre VI Etude spécifique sur le devenir de
deux désinfectants largement utilisés dans les hôpitaux :
l'hypochlorite de sodium et le glutaraldehyde
Table 3. Microbiological and toxicological
characterizations of the mixture samples
Parameters
|
Units
|
Means
|
Minima
|
Maxima
|
SD
|
n
|
Fecal coliforms
|
MPN
|
|
<3
|
2 400
|
|
4
|
EC50 Vibrio fischeri
|
TU
|
1.42
|
1.1
|
1.54
|
0.25
|
4
|
(MICROTOX -- 5mn)
|
|
|
|
|
|
|
EC50 Vibrio fischeri
|
TU
|
3.4
|
2.47
|
4.15
|
0.7
|
4
|
(MICROTOX -- 15mn)
|
|
|
|
|
|
|
EC50 Daphnia
|
TU
|
44
|
9.8
|
116.8
|
34
|
8
|
11.6. Discussion
11.6.1. Relationship between COD and TOC
Studies on hospital wastewater reported that these effluents
are essentially domestic (i.e. sanitary wastewater from residential and
commercial sources) and are characterized by pollutant concentrations of
BOD5, COD, TSS and TOC (EPA, 1989b; Mansotte and Justin, 2000).
BOD5 value was not analyzed in this study. In table 4 the results
obtained for the hospital effluents were compared with the approximate ranges
for domestic wastewater reported in the literature.
TOC and COD concentrations are greater than the values
proposed by Metcalf and Eddy (1991) for domestic wastewater. COD and TOC were
reacted to completion (Gray and Becker, 2002), and a good linear correlation
was found between them in the results of this study (r = 0.95, r2 =
0.9, DurbinWatson statistic DW = 1.96, P = 0.0039) the graph is presented in
figure 2.
Table 4. Comparison between domestic wastewater and
hospital effluents
Parameters Units Approximate ranges of Approximate ranges of
Results of the study
domestic and hospital domestic wastewater on hospital
wastewater (Metcalf & Eddy, 1991b) wastewater
(EPA, 1989b)
Chlorides
|
mg/L
|
|
30 - 100
|
63 - 359
|
TSS
|
mg/L
|
60 - 200
|
100 - 350
|
155 - 298
|
COD
|
mg/L
|
150 - 800
|
250 - 1000
|
362 - 1492
|
TOC
|
mg/L
|
50 - 300
|
80 - 290
|
160 - 350
|
BOD5
|
mg/L
|
50 - 400
|
110 - 400
|
|
Chapitre VI Etude spécifique sur le devenir de
deux désinfectants largement utilisés dans les hôpitaux :
l'hypochlorite de sodium et le glutaraldehyde
|
400 350 300
|
|
y = 0.1707x + 85.10
|
|
|
|
|
|
|
2 a)
|
250
|
|
r= 0.95
|
|
|
|
|
|
|
|
E
|
200
|
|
|
|
|
|
|
|
|
|
c.) 0 1--
|
150
|
|
·
|
|
·
|
|
|
|
|
|
|
100
|
|
|
|
|
|
|
|
|
|
|
50
|
|
|
|
|
|
|
|
|
|
|
0
|
|
|
|
|
|
|
|
|
|
|
I
|
I
|
I
|
|
I
|
I
|
I
|
I
|
I
|
0 200 400 600 800 1000 1200 1400 1600
COD (mg/L)
Figure 2 : Linear regression between TOC and COD
concentrations
Information reported in the literature assume a COD/TOC of
ratio 3 frequently found in many wastewater (Seiss et al., 2001). Gray and
Becker (2002) reported a semi-empirical equation to determine the ratio between
COD expressed in mg 02/L and TOC in mg C/L.
COD = 2.67 TOC (12)
The variations of COD, TOC and the COD/TOC ratio in the
samples of ITDD hospital wastewater, for the 6 first days of sampling, are
depicted in figure 3. The COD/TOC ratio found in hospital wastewater range from
2.01 to 4.26. The difference could be an effect of the presence of inorganic
substances in COD concentrations. The biodegradability of organic substances is
a measure of the speed and completeness of its biodegradability by
microorganisms (Sponza, 2003), and therefore the BOD5/COD and
COD/TOC ratios could be used to analyze the difficulty or not for organic
substances to be degraded. In this study, the precise biochemistry knowledge of
hospital wastewater, necessary to analyze the biodegradability by global
parameters ratio, is not completed, because BOD5 was not carried
out. However, the data obtained from COD/TOC ratio, when compared with
information reported in the literature on this issue, can be ranged in two
groups: (i) COD/TOC from 2.01 to 3.00, and (ii) COD/TOC from 3.01 to 4.26. In
the first group the organic substances would be degraded by microorganisms with
out difficulties, but in the second group the substances would be difficult to
degrade. In the future, it will be necessary to verify this analysis by
comparing the COD/TOC ratio with the BOD5/COD ratio.
Chapitre VI Etude spécifique sur le devenir de
deux désinfectants largement utilisés dans les hôpitaux :
l'hypochlorite de sodium et le glutaraldehyde
4,50
- 4,00
- 3,50
- 3,00 o
- 2,50
- 2,00
- 1,50 o c.)
- 1,00
- 0,50 0,00
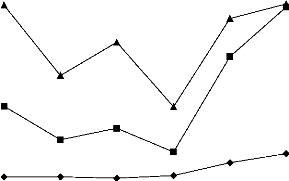
1600
1400 -
0 c
· 1200 -
fa L.
m 1000 -
ta
c
m :1..*
u ci) 800 -
m E
0
1- 600 - -o
c ta
o 400 -
O
200 -
1 2
-3- COD mg/I - ·- TOC mg/L -A-COD/TOC ratio
0
Figure 3: Variations of COD, TOC and COD/TOC ratio in
hospital effluents of ITDD
11.6.2. Influence of chloride on AOX formation and
toxicity on aquatic organisms
Organic halogen compounds are formed by reaction of chlorine
with organic wastewater compounds. The hypothesis that sodium hypochlorite,
used as elementary chlorine in disinfections of hospital wastewater of ITDD, is
responsible for the disinfecting effect of AOX on aquatic organisms seems to be
verified. In fact, the major mass carriers for the AOX in hospital effluents
are most likely iodized Xray contrast media, solvents, disinfectants, cleaners
and drugs containing chlorine. In general, the maximum contribution of drugs to
the AOX is not above 11% (Kümmerer et al., 1998). Beyond that it is also
known that the AOX concentration in the urine of patients not treated with
drugs is very low. It is normally between 0.001 to 0.2 mg/L (Schulz and Hahn,
1997). Due to the dilution effect, no substantial contribution from this source
is consequently expected (Kümmerer, 2001).
Previous studies on AOX presence in wastewater explained the
formation of this "non-conventional" pollutants by the presence of
organochlorine compounds (Seiss et al., 2001; Lôpez, 2003), and by the
oxidation of bromide by hydroxyl radicals (von Gunten and Oliveras, 1998) or by
the oxidation of iodized X-ray contrast media (Sprehe et al., 2001). Brominated
organic compounds are negligible for the AOX in the hospital effluents
(Kümmerer, 2001). The conditions of sampling taken for this study, i.e.
choice of a wastewater collector which does not receive iodized X-ray contrast
media effluents
Chapitre VI Etude spécifique sur le devenir de
deux désinfectants largement utilisés dans les hôpitaux :
l'hypochlorite de sodium et le glutaraldehyde
form radiography department, lead to the conclusion that the
AOX formation, in hospital wastewater from the infectious and tropical diseases
department, is mainly due to the influence of sodium hypochlorite (chlorine).
The lower chloride concentrations correspond to lower concentration of
reduction of AOX formation. The effluent samples showing the higher chloride
concentration were the same generating the higher AOX results and the most
important acute toxicity on Daphnia magna.
11.6.3. Acute toxicity of physicochemical parameters of
the ITDD effluents on the aquatic organisms
Fecal coliform populations of ITDD wastewater were affected
because chloride values of hospital wastewater are greater than values
generally uncounted in domestic wastewater. Although, hospital use and
discharge (into sewer network) large amounts of water (U.S. EPA, 1989b; Leprat,
1998; Emmanuel et al., 2002) which allows the dilution of higher pollutant
concentrations to lower values, it seems necessary to follow-up the behavior of
microbial populations of urban wastewater treatment plants which receive these
hospital effluents containing higher chloride and AOX concentrations.
Each of the two species responded differently to hospital
wastewater samples. Acute toxicity on marine organism was performed using the
MICROTOX test to study the inhibition of the bacteria bioluminescence, and
acute toxicity on freshwater zooplankton was studied using Daphnia magna
bioassay.
The ITDD effluents had an inhibition on the bacteria
bioluminescence. It is difficult to attribute this toxicity to one or all the
determined physicochemical parameters. The variation of toxicity on Vibrio
fischery in all the samples did not have good correlations with chloride
and AOX concentrations. Because the assay was realized only under 5 and 15 min
of exposure, it seems that the toxic effects, of organochlorine compounds
containing in hospital wastewater samples, were not completed. The sodium
hypochlorite needs a contact time of 20 minutes to inactivate bacteria.
Although in all cases the pH values in the samples were ranged from 7.9 to 8.8,
the exposure time lower than 20 minutes is probably the cause of absence of a
good correlation among the toxicity and the physicochemical concentration.
Beside the pH conditions, it seems that 30 minutes of exposure time is the
ideal contact time to evaluate toxicity of organochlorine containing in
hospital wastewater using MICROTOX test.
Although the results of AOX concentrations in some cases were
lower than the discharge regulation, the results of toxicity test of hospital
wastewater on Daphnia magna indicated potential toxicity. Ail the
samples demonstrated the presence of an acute toxicity of hospital effluents on
aquatic organisms. A good linear regression was found between EC50
results of toxicity on Daphnia magna and AOX assessed with r = 0.98,
r2 = 0,95, DW = 1.13, P = 0,0001 (figure 4). No significant
difference was observed between the EC50 Daphnia -- AOX
correlation and the EC50 Daphnia -- chlorides correlation
(r = 0.98, r2 = 0,95, DW = 1.75, P = 0,0001). The variations of
EC50 (UT)
Chapitre VI Etude spécifique sur le devenir de
deux désinfectants largement utilisés dans les hôpitaux :
l'hypochlorite de sodium et le glutaraldehyde
Daphnia, pH, chlorides and AOX concentrations of ITDD
hospital wastewater are highlighted in figure 5.
The weight of TSS on the most toxic sample (EC50 of
116.8 TU) was studied by passing sample through Giving a 0.45 pm pore size
membrane and performing the Daphnia magna bioassay. The 24h
EC50 of filtered sample was 29.7 TU. It seems that TSS contributed
in increasing toxicity of hospital wastewater on Daphnia magna
approximately at 74.57%. Because, the application of NaOCI as elementary
chlorine was made before the sedimentation and filtration processes of
effluents, it allowed the formation of organochlorine compounds such as AOX
resulting from chemical reaction between the organic matters in wastewater an
chlorine.
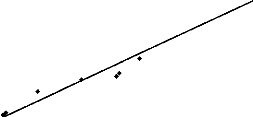
140 --
120 --
ea
.2 100 -- .c
o. as
0
80--
I-
60--
I4 Cà
Lu 40 --
20 --
·
y = 115.54x - 36.115 r= 0.98
0 0,2 0,4 0,6 0,8 1 1,2
AOX (mg/L)
Figure 4: Linear regression between EC50
(UT) Daphnia and AOX concentrations
The results of this study on toxicological effects of NaOCI
and its contribution to AOX formation in hospital wastewater allows to conclude
that chloride could be used as indicator of the toxicity of hospital wastewater
on aquatic organisms when NaOCI was used in elementary chlorine of wastewater
collector which does not receive effluents from hospital radiography
department. It seems necessary to verify in other studies, these first
observations on toxicity of hospital effluents.
Chapitre VI Etude spécifique sur le devenir de
deux désinfectants largement utilisés dans les hôpitaux :
l'hypochlorite de sodium et le glutaraldehyde
400 -
-c ·
c 350 -
-- u) · --I k ' I
Cle a)
300 - /
· E / \
o I
2 250 - /
/
\
u 0 / \
d (, , `
\\
c -- .- · /I
.-- 1.- 200 -
.c c .. /
Q. CD /
u
.
0ta
c o 150 -
\ li \\
P u
\\ ik \
m x im.
- 0 100 - , . ..
(0 ..e.
.. . /
-Ir
|
. .
./
|
)3'
, ·
|
_ ..*
|
-
|
-9 -8 -7 -6 -5 -4 -3 -2 -1
10
o.
|
0
1 2 3 4 5 6 7 8
Semples
- a- Chlorides mg/L Daphnie UT - pH unit -m-AOX mg/L
Figure 5: Variations of EC50 (UT)
Daphnia, pH, chlorides and AOX concentrations of ITDD hospital
wastewater
11.7. Conclusion
The results of this study showed that the application of NaOCI
in wastewater can reduce bacteria water pollution and give rise to toxicity
effects on aquatic organisms. Low MPN ranging from <3 to 2400 for 100 mL
were detected for fecal coliforms. Toxicity test using MICROTOX showed the
toxicity of hospital wastewater on Vibrio fischeri, however bad
correlations were observed between this toxicity and the concentrations of
chloride and AOX. It seems that 30 minutes of exposure time is the ideal
contact time to evaluate toxicity of organochlorine containing in hospital
wastewater using MICROTOX test. The results of 24-h EC50 on
Daphnia magna demonstrated the high toxicity effect of hospital
wastewater on these species. Some good correlation were observed between these
toxicity results and the concentrations of chloride and AOX.
The identification of an ideal concentration of NaOCI in
disinfecting hospital wastewater, i.e. its NOEC (Non Observed Effect
Concentration) on algae and Daphnia magna, seems to be a research
issue which could facilitate the control of AOX toxicity effects on aquatic
organisms. Therefore, it would be necessary to follow-up at various dosages the
biocide properties of NaOCI on fecal coliforms and its toxicity effects on
aquatic organisms.
Chapitre VI Etude spécifique sur le devenir de
deux désinfectants largement utilisés dans les hôpitaux :
l'hypochlorite de sodium et le glutaraldehyde
References
Abe T., Saito H., Niikura Y., Shigeoka T., Nakano Y.
Embryonic development assay with Daphnia magna
application to toxicity of analine derivatives. CHEMOSPHERE,
45:487-495, 2001.
Addinsoft. Aide XlstatPro, XLStat version 5.0 (b8.3).
Copyright 2000-2001, Addinsoft, Paris; 2001. AFNOR (Association
Française de Normalisation). Qualité de l'eau, tome 2 :
méthodes d'analyse 1, analyse organoleptique, mesures physico-chimiques,
paramètres globaux, composés organiques. Recueil de normes
françaises 1997, 2e édition, Paris; 1997a.
AFNOR (Association Française de Normalisation).
Qualité de l'eau, tome 3 : méthodes d'analyse 2,
éléments majeurs, autres éléments et
composés minéraux. Recueil de normes françaises 1997,
2e édition, Paris; 1997b.
AFNOR (Association Française de Normalisation).
Qualité de l'eau, tome 4 : méthodes d'analyse 3, analyse
biochimique et biologique, analyse microbiologique, textes
réglementaires. Recueil de normes françaises 1997, 2e
édition, Paris; 1997c.
AQUIRE (Aquatic Information Retrieval). EPA ERL-Duluth's
Aquatic Ecotoxicology Data Systems. U.S. EPA (United States Environmental
Protection Agency), Duluth, MN; 1994.
ASTM (American Society for Testing and Materials).
Standard guide for conducting renewal life-cycle toxicity tests with
Daphnia magna. In.: Annual Book of ASTM Standards, E
1193-94. ASTM, Philadelphia, 1994.
Banerjee S., Howard P.H., Rosenberg A.M., Dombrowski
A.E., Sikka H., Tulis D.L. Development of a general kinetic model for
biodegradation and its application to chlorophenols and related compounds.
Envron Sci Technol, 18:416-422; 1984.
Baxter G. Chlorine disinfection -- the industry standard.
In.: International Speciazed Conference: Disinfection of Potable Water. Kruger
National Park, South Africa, 1998.
Bernet S., Fines M. Effluents du CHU de CAEN : Etude
qualitative et quantitative de la flore microbienne et recherche de
bactéries multirésistantes. Poster.
Quatrième journée du Réseau Régional
d'Hygiène de Basse-Normandie, Caen, 2000.
Boyce S.D., Hornig J.F. Reaction pathways of
trihalomethane formation from the halogenation of dihydroxyaromatic model
compounds for humic acid. Environ. Sci. Technol., 17:202-211, 1983. Brondeau
M.T., Falcy M., Jargot S., Miraval S., Protois J.C., Reynier M., Scheneider O.
Eaux et extraits de Javel. Fiche toxicologique N° 157 de l'INRS. Cahiers
de notes documentaires -- Hygiène et sécurité du travail,
178:11-15, 2000.
Bulich A.A. Use of luminescent bacteria for determining
toxicity. In.: ASTM STP 667 Aquatic Toxicity, Philadelphia, 1979.
Carey J., Cook P., Giesy J., Hodson P., Muir D., Owens
W., Solomon K. Ecotoxicological risk assessment of the chlorinated organic
chemical. In.: Carey J., Cook P., Giesy J., Hodson P., Muir D., Owens W.,
Solomon K., (ed) Workshop on Environmental Risk Assessment for Organochlorine
Compounds. SETAC (Society of Environmental Toxicology and Chemistry),
Pensacola-Florida, 1998.
Chapitre VI Etude spécifique sur le devenir de
deux désinfectants largement utilisés dans les hôpitaux :
l'hypochlorite de sodium et le glutaraldehyde
CCLIN (Comité de Coordination de Luttes contre
les Infections Nosocomiales). Élimination des effluents liquides des
établissements hospitaliers -- Recommandations. Institut
Biomédical des Cordeliers, Paris, 1999.
Cooney J.D. Freshwater tests. In.: Rand G.M. (Ed.).
Fundamentals of aquatic toxicology: Effects, environmental fates and risks
assessment. Second ed., Taylor & Francis, Washington DC, 1995.
Deloffre-Bonnamour N. Les rejets des établissements de santé :
des effluents liquides aux déchets solides. Mémoire de
Maîtrise, Université Claude Bernard-Lyon1, Institut Universitaire
Professionnalisé, Génie de
l'Environnement--Ecodéveloppement, Lyon; 1995.
Emmanuel E., Blanchard J.-M., Keck G., Perrodin Y.
Caractérisation chimique, biologique et écotoxicologique des
effluents hospitaliers. Déchets Sciences et Techniques, revue
francophone d'écologie industrielle, 22:31-33; 2001.
Emmanuel E., Perrodin Y., Keck G., Blanchard J-M.,
Vermande P. Effects of hospital wastewater on aquatic ecosystem. Proceedings of
the XXVIII Congreso Interamericano de Ingenieria Sanitaria y Ambiental. Cancun,
México, 27-31 de octubre, 2002. CDROM.
Erlandsson B., Matsson S. Medically used radionucides in
sewage sludge. Water, Air, and Soil Pollution, 2:199-206; 1978.
European Commission. Directive 98/15/EEC amending Council
Directive 91/271/EEC: Urban wastewater treatment. Brussels; 1998.
Finney D.J. Probit analysis. 3rd ed. Cambridge
Univ. Press, Cambridge, 1971.
Gartisser St., Brinkler L., Erbe T., Kümmerer K.,
Willmund R. Contamination of hospital wastewater with hazardous compounds as
defined by § 7a WHG. Acta hydrochim. Hydrobio 24, 2:90-97; 1996. Gray
S.R., Becker N.S.C. Contaminant flows in urban residential water systems. Urban
water, 4:331346; 2002.
Halling-Sorensen B. Occurrence, fate and effects of
pharmaceutical substances in the environnement -- A review. CHEMOSPHERE,
36:357-393; 1998.
Hanazato T. Growth analysis of Daphnia early
juvenile stages as an alternative method to test the chronic effect of
chemicals. Chemosphere, 36:1903-1909; 1998.
Jehannin P. Caractérisation et gestion des
rejets liquides hospitaliers -- Etude particulière de la situation du
C.H. de Hyères (Var). Mémoire de fin d'études, Ecole
Nationale de la Santé Publique, Rennes; 1999.
Jokela J.K., Eloma E., Salinoja-Salonen M.S. Adsorbable
organic halogens (AOX) in drinking water and aquatic environment in Finland. J
Water Supply Res. Technol, 41:4-11, 1992.
Jolibois B., Guerber M., Vassal S. Glutaraldehyde in
hospital wastewater. Arch. Environ. Contam. Toxicol. 42:137-144;
2002.
Kinnersley D. Discharge consent and compliance policy: a
blueprint for the future. NRA (National Rivers Authority) Water Quality Series,
No. 1, Bristol; 1990.
Kümmerer K. Drugs in the environment : emission of
drugs, diagnostic aids and disinfectants into wastewater by hospitals in
relation to other sources -- a review, Chemosphere 45:957-969;
2001.
Chapitre VI Etude spécifique sur le devenir de
deux désinfectants largement utilisés dans les hôpitaux :
l'hypochlorite de sodium et le glutaraldehyde
Kümmerer K., Meyer M., Steger-Hartmann T.
Biodegradability of the anti-tumour agent Ifosfamide and its occurrence in
hospital effluents and communal sewage. Wat. Res., 11:2705-2710, 1997.
Kümmerer K., Gartiser St., Erbe T., Brinkler L. AOX-emissions from
hospital into municipal wastewater. Chemosphere, 36:2437-2445,
1998.
Leprat P. Les rejets liquides hospitaliers, quels agents
et quelles solutions techniques ? Troisième journée du
Réseau Régional d'Hygiène de Basse-Normandie, Caen,
1998.
Lopez A., Mascolo G. Ciannarella, Tiravanti G. Formation
of volatile halogenated by-products during chlorination of isoproturon aqueous
solutions. Chemosphere, 45:269-274; 2001.
Lôpez M.C.C. Determination of potentially
bioaccumulating complex mixtures of organochlorine compounds in wastewater: a
review. Environment Int. 28:751-759; 2003.
Mansotte F., Jestin E. Les rejets liquides des
établissements de santé : Caractérisation à la
source et impact sur l'environnement marin côtier. Direction
Départementale des Affaires Sanitaires et Sociales de la Seine Maritime,
Agence de l'Eau de la Seine Normandie, Nanterre, 2000.
Marhaba T.F., Washington M.B. Drinking water disinfection
and by-products: history and current practice. Adv. Environ. Res. 2:103-115;
1998.
MATE (Ministère de l'aménagement du
territoire et de l'environnement). Arrêté du 2 février 1998
relatif aux prélèvements et à la consommation d'eau ainsi
qu'aux émissions de toute nature des installations classées pour
la protection de l'environnement soumises à autorisation. 3.0 (Journal
Officiel) n° 52 du 3 mars 1998 page 3247, Paris.
Metcalf & Eddy, Inc. Wastewater Engineering:
Treatment, disposai, and reause. 31t1 ed. Revised by Tchobanoglous
G., Burton F.L. Irwin/McGraw-Hill, New York, 1991.
NTP (National Toxicology Program). Toxicology and
carcinogenesis studies of chlorinated water (CAS NOS 7782-50-5 and 7681-52-9)
and chloraminated water (CAS No. 10599-90-3) (Deionized and charcoal-filtrered)
in F344/N rats and B6C3F1 mice (drinking water studies). U.S. Dept. of Health
and Human Services, National Institutes of Health, Research Triangle Park, NC,
1992; NTP TR 392.
OECD (Organization for Economic Cooperation and
Development). Daphnia sp. Acute immobilization test and reproduction
test. In. : OECD Guidelines for testing of chemicals, 211. OECD, Paris; 1984.
Perrodin Y. Proposition méthodologique pour l'évaluation de
l'écotoxicité des eflluents aqueux : Mise au point d'un
Multi-Test Macroinvertébrés (M.T.M.) -- Application aux lixiviats
de décharges et à leurs composants caractéristiques.
Thèse de doctorat. Institut National des Sciences Appliquées de
Lyon; 1988.
Richardson M. L., Bowron J. M. The fate of pharmaceutical
chemicals in the aquatic environment. J. Pharm. Pharmacol. 37:1-12;
1985
Salinoja-Salonen M.S., Jokela J.K. Measurement of organic
halogen compounds in urine as an indicator of exposure. Scand J Workplace
Environ Health, 17 :75-78; 1991.
Schowanek D., Fox K., Holt M., Schroeder F., Koch V.
GREAT-ER: a new tool for management and risk assessment of chemicals in river
basin. Water Sci. Technol. 43:179-185, 2001.
Seiler H.G., Sigel H., Sigel A. Handbook on toxicity of
inorganic compounds. Marcel Dekker, Inc., New York, 1988.
Chapitre VI Etude spécifique sur le devenir de
deux désinfectants largement utilisés dans les hôpitaux :
l'hypochlorite de sodium et le glutaraldehyde
Schulz S., Hahn H.H. Der kanal als reactor-undersuchugen
zur AOX-Bildung durch wirkstoffe in reinigunsmittenln. gwf Wasser Abwasser,
138:109-120; 1997.
Seiss M., Gahr A., Niessner R. Improved AOX degradation
in UV oxidative wastewater treatment by dialysis with nanofiltration membrabe.
Wat. Res., 13:3242-3248; 2001.
Sponza D.T. Application of toxicity tests into discharges
of the pulp-paper industry in Turkey. Ecotoxicology and Environmental Safety,
54:74-86; 2003.
Sprehe M., GeiBen S.-U., Vogelpohl A. Traitement of
AOX-containing wastewater from hospitals -- Degradation of iodized X-ray
contrast medium. Korrespondenz Abwasser, 46, 4:548-558; 1999.
Sprehe M., GeiBen S.-U., Vogelpohl A. Photochemical
oxidation of iodized X-ray contrast media (XRC) in hospital wastewater. Water
Science and Technology, 5:317-323; 2001.
Stokinger H.E. The halogens and the nonmetals boron and
silicon. In: Clayton G.D., Clayton F.E., Eds. Patty's industrial hygiene and
toxicology, 3`l ed., Vol 2B., John Wiley & Sons, New York;
1981.
U.S. EPA (United States Environmental Protection
Agency) Drinking water criteria document for chlorine, hypochlorous acid and
hypochlorite ion. Environmental Criteria and Assessment Office, U.S. EPA,
Cincinnati, OH; 1989a.
U.S. EPA (United States Environmental Protection Agency)
Chemical summary for chlorine. Office of pollution prevention and toxics, U.S.
EPA, Cincinnati, OH; 1994a. EPA 749-F-94-010a.
U.S. EPA (United States Environmental Protection Agency)
Reportable quantity document for chlorine, draft. Environmental Criteria and
Assessment Office, U.S. EPA, Cincinnati, OH; 1994b.
U.S. EPA (United States Environmental Protection
Agency) Preliminary data summary for the hospitals point source category.
Office of water regulations and standards, Office of water, U.S. EPA,
Washington, D.C., 1989b. EPA 440/1-89/060-n.
Verschueren K. Handbook of environmental data on organic
chemicals. Third ed., Van Nostrand Reinhold Company Inc., New York,
1996.
Von Gunten U., Hoigné J. Bromate formation through
ozone and hydroxyl radicals. In.: Minear R.A., Amy G.L. (Eds.) Desinfection
by-products in water treatment. Lewis Publisher, Boca Raton, 1995.
Von Gunten U., Oliveras Y. Advanced oxidation of bromide
containing waters: bromate formation mechanism. Environ. Sci. Technol.
332:63-70, 1998.
White G.C. Handbook of chlorination. 2nd edition, Van
Nostrand Reinhold Company Inc., New York;
1986.
Chapitre VI Etude spécifique sur le devenir de
deux désinfectants largement utilisés dans les hôpitaux :
l'hypochlorite de sodium et le glutaraldehyde
|