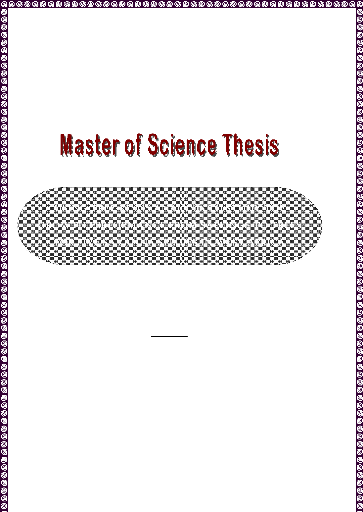
UNIVERSITY OF ABOMEY-CALAVI (BENIN)
****************
FACULTY OF AGRONOMIC SCIENCES
(FSA)
************
Department of Natural Resource
Management (AGRN)
TOPIC
Submitted by Franck Cocou A. SINSIN
Promoters
Prof. Dr. Ir Brice A. SINSIN (University of
Abomey-Calavi, Benin)
Prof. Dr. Stefan POREMBSKI (University of
Rostock, Germany)
Dr Bettina ORTHMANN (University of Rostock,
Germany)
Defended 6th January 2009
TABLE OF CONTENTS
TABLE OF CONTENTS i
LIST OF FIGURES iii
LIST OF PICTURES iv
LISTE OF TABLE iv
DEDICATION v
ACKNOWLEDGEMENTS vi
TECHNICAL EXPRESSIONS vii
Abstract ..viii
Résumé ix
1. Introduction 1
1.1. Background on tree dating methods 1
1.2. The interest of the study 2
1.3. The aims 3
1.4. Research questions 3
2. Study area 3
3. Materials and methods ..5
3.1. Studied plant species and samples collection 5
3.2. Sample processing ..9
3.3. Method used to study the wood anatomy .10
3.4. Method used to study the growth performances 10
3.5. Cross-dating 11
3.6. Method used to study the fire regime 12
3.7. Nature of data 12
4. Results 13
4.1. Wood anatomy of different species studied 13
4.1.1. Anogeissus leiocarpa 15
4.1.2. Detarium microcarpum .16
4.1.3. Diospyros abyssinica 17
4.1.4. Isoberlinia doka 17
4.1.5. Pterocarpus erinaceus .18
4.1.6. Conclusion on wood anatomy 19
4.2. Age and growth performances for investigated species 19
4.2.1. Age, ring increment and cross-dating 19
4.2.2. Growing in height .23
4.2.3 Conclusion on tree species growth 24
4.3. Fire regime analysis from tree-rings of investigated
species... 24
4.3.1. Fire master chronology in CNP and UAC 24
4.3.2. Fire interval 25
4.3.3. Fire frequency and intensity in Comoé national Park
and Upper Aguima Catchment 27
4.3.4. Conclusion on events of fire in savanna areas . 27
5. Discussion 28
Conclusion 29
6. Literature cited 30
Appendix
LIST OF FIGURES
Figure 1 Mean monthly rainfall for studied sites
(CNP & UAC)
Figure 2 Macroscopically polished stem discs
observation of investigated species Figure 3 The different
parts of wood. Stem disc of D. microcarpum
Figure 4 The common wood anatomical legend
Figure 5 Wood anatomy of A. leiocarpa
(Combretaceae)
Figure 6 Wood anatomy of D. microcarpum
(Caesalpiniaceae)
Figure 7 Wood anatomy of D. abyssinica
(Ebenaceae)
Figure 8 Wood anatomy of I. doka
(Caesalpinaceae)
Figure 9 Wood anatomy of P. erinaceus
(Fabaceae)
Figure 10 Cross-dating ring width of
Diospyros abyssinica oldest sampled tree cut at two different
heights
Figure 11 Cross-dating ring width of
Detarium microcarpum oldest sampled tree cut at two different
heights
Figure 12 Cross-dating ring width of one of
sampled tree of Anogeissus leiocapus cut at two different heights
Figure 13 Initial height growth for some of
investigated species
Figure 14 Fire history in Comoé National
Park
Figure 15 Fire history in Upper Aguima
Catchment
Figure 16 Example of fire scars on stem discs
Figure 17 Illustration of compartmentalisation
concept on D. microcarpum stem disc
LIST OF PICTURES
Picture 1 Morphological characteristics of
Anogeissus leiocarpa Picture 2 Morphological
characteristics of Detarium microcarpum Picture 3
Morphological characteristics of Diospyros abyssinica
Picture 4 Morphological characteristics of
Isoberlinia doka Picture 5 Morphological
characteristics of Pterocarpus erinaceus Picture 6
Some of the analysed stem discs
Picture 7 The used electronic forest compass
Picture 8 The used polishing machine
Picture 9 The Lintab combined to microscope for
measurement
LIST OF TABLES
Table 1 The five tree species investigated in
two different study sites
Table 2 Some characteristics of investigated
species
Table 3 Age and MRI of the investigated
species
DEDICATION
This work was funded by the BIOTA Africa
programme (Biodiversity Monitoring Transect Analysis in Africa). It
was mainly realized with the technical support of Institute of Agronomy
in the Tropics (University of Göttingen) where I analysed my
data, the Laboratory of Applied Ecology (University of
Abomey-Calavi, Benin) and the Laboratory of Biosciences
(University of Rostock, Germany).
ACKNOWLEDGEMENTS
«My people are destroyed from lack of knowledge...»
Hosea 4:6 (NIV).
The above biblical excerpt from the prophet Hosea moved me
into action to acknowledge the promoters of this work. My warmest thanks are
due in the first place to my professors who have shown the very greatest spirit
of co-operation and whose competence, understanding and helping have made my
first experience on dating of tropical trees species a successful issue.
In this respect, special mention is made of the invaluable
contribution of Professor Brice Sinsin, head of Laboratory of
Applied Ecology from the University of Abomey -Calavi (Benin) who played a key
role in the achievement of this thesis. I could not have achieved this alone
without his help. He accepted me into his lab as a postgraduate student and
showed me the way to take. His advices and recommendations enabled me to
overcome successfully. I must acknowledge him with deepest gratitude for the
excellent advice, encouragement and guidance.
I am also particularly indebted to Professor Stefan
Porembski and his assistant Dr. Bettina Orthmann from
the University of Rostock (Germany) who accepted me into their laboratory and
taught me the methods of tree dating. Dr Bettina was never far from me. First,
she facilitated the process of my trip. Secondly, she introduc ed me to the
specialist of tree-ring dating, helped me to improve my research proposal and
offered me a pleasant working time. Thirdly, she read the entire work and
helped me once more to improve it. I miss words to tell her my acknowledgements
as she deserves.
I should express my respectful thanks in special place to
PD Dr Martin Worbes for his simplicity, helpful and teaching
during the two months I worked with him. I got tree-ring dating in his lab.
I could never forget Dr Romain Kakaï
Glèlè, Dr Klaus Hennenberg, Miss Sofie Blanchart
and a very large number of my graduate colleagues (Promotion 31) for
the fruitful exchanges.
Finally, I have to render my sincere thankfulness to my family
and Miss Camen T. Adjadémè whose fondness and
love helped me to bring to a successful conclusion.
TECHNICAL EXPRESSIONS
Bark: All the tissues outside the cambium that
protects the trunk and all
branches
Callous margin: Edge of callous tissue
overgrowing a stem wound
Thin layer of meristematic tissue that lies between, and gives
rise by Cambium: active division to, secondary xylem on the
inside and secondary phloem on the outside
Compartmentalisation: A dynamic defence process
in an injured tree which forms structural and chemical boundaries in order to
resist the spread of pathogens
Cross-dating: The procedure of matching
variations in ring width or other ring
characteristics among several tree ring series, allowing the
identification of the exact year in which each tree ring was formed, or th e
geographic origin of the tree
Innermost ring: First formed ring, situated
immediately next to pith
Master fire chronology: Chronologies of fire
dates constructed from the fire scar information derived from all samples
within a collection area
Outermost ring: The most recently formed tree
ring still visible on a wood sample
Parenchyma: Mostly rectangular, storage cells
alive in the sapwood, with simple
pits, present in the rays and axially oriented in xylem and
phloem
Parenchyma band: Parenchyma forming one or more
tangential lines within a tree ring
Pith: The central core of a stem and of twigs,
consisting of storage
tissue (parenchyma) with unlignified cell walls
Radius: On a stem disc, measuring line between
the pith and the outermost ring
Stem disc: Cross-wood section
ABSTRACT
Dendrochronology was used to study the wood anatomy and growth
performance of five West African savanna species namely Anogeissus
leiocarpa (DC.) Guill., Perr., Detarium microcarpum Guill.,
Perr., Diospyros abyssinica (Hiern) F. White, Isoberlinia doka
Craib., Stapf., and Pterocarpus erinaceus Poir. This technique
was also used to reconstruct the fire past in studied areas that are
Comoé National Park in Côte d'Ivoire and Upper Aguima Catchment in
Benin. Tree-ring analysis (Dendrochronology) through 72 stem discs collected
from two to three different heights of trees and using cross dating approach
helped to better understand the biology of wood. Using these data, we
categorized the five s pecies in different groups according to their wood
anatomy and radial growth performance. Then, we determined, described the inter
and intra specific variation of the growth rate. Finally, we compared the two
studied areas among their seasonal fire interva l, its frequency and intensity.
So, species such as Detarium microcarpum Guill., Perr., and
Isoberlinia doka Craib. were identified as fast growing species with
brown wood. The rings boundaries were easily distinguished and formed by
marginal parenchyma ba nds. Wood of Anogeissus leiocarpa (DC.) Guill.,
and Pterocarpus erinaceus Poir. were white or yellow in colour. The
rings showed a variation in vessels distribution and the growth performances
were also good. Diospyros abyssinica (Hiern) F. White was demonstrated
like a slow-growing species that wood's colour usually varies between white and
yellow with black streaks. The rings are narrow and not identifiable
macroscopically. However, they were easily measured with high accuracy. The
rings boundaries were presented in single concentric line and characterized by
patterns of alternating parenchyma and fibre bands. Finally, both studied sites
were seriously disturbed by using of several consecutive fires that caused the
injuries in tree and ecosystems.
Key words: Tree-dating, Dendrochronology, wood
anatomy, Bush fire, Savanna, Upper Aguima Catchment (Benin), Comoé
National Park (Côte d'Ivoire)
RESUME
La présente étude a porté sur
l'utilisation de la dendrochronologie (Analyse des cernes d'accroissement) pour
comprendre l'anatomie et le rythme de croissance de cinq espèces
végétales caractéristiques de la région savanicole
de l'Afrique de l'Ouest. Cet outil a été également
utilisé à partir de la provenance du matériel
d'étude pour retracer l'historique des feux de végétation
dans cette région et précisément dans le Parc National de
Comoé en Côte d'Ivoire et dans la forêt claire des Monts
Couffé du Bénin. Les objectifs poursuivis ont été
de documenter en premier lieu l'anatomie du bois pour les espèces
Anogeissus leiocarpa (DC.) Guill., Perr., Detarium microcarpum
Guill., Perr., Diospyros abyssinica (Hiern) F. White,
Isoberlinia doka Craib., Stapf., et Pterocarpus erinaceus Poir.
Dans un second temps, il fallait déterminer l'âge des arbres
échantillonnés par l'analyse des cernes puis étudier la
variabilité des performances de croissance entre ces différentes
espèces. Enfin, il était question d'utiliser la méthode
pour fournir des informations sur l'écologie et la dynamique des
habitats de ces espèces, en particulier, l'impact des feux de
végétation sur la biodiversité. La méthode
utilisée est basée essentiellement sur l'observation à
l'oeil nu, à la loupe, au microscope et au scanner des cernes
d'accroissement qui ont été comptés utilisant la technique
«cross da ting» via le logiciel TSAPWin à partir des rondins
de bois coupés à deux différentes hauteurs. L'anatomie du
bois a pu être reconstituée par une combinaison d'outils :
microscope associé au Lintab avec le logiciel Leica et parfois le
scanner. Les résultats obtenus ont permis de catégoriser les
espèces suivant les caractéristiques anatomiques et les
performances de croissance. Ainsi les espèces comme D. microcarpum
et I. doka sont identifiées comme des espèces
à bois bruns présentant des cernes dont les limi tes sont
facilement observables à l'oeil nu et caractérisées par
une bande de parenchyme marginale. Les arbres de ces espèces ont une
croissance relativement bonne. A. leiocarpa et P. erinaceus
sont des espèces à bois variant entre le blanc et le jaune
et présentant des cernes avec une variation dans la distribution des
vaisseaux. Les performances sont relativement assez bonnes. D. abyssinica
présente des cernes rétrécies, difficilement
identifiables aussi bien à l'oeil nu qu'à la loupe. Cependant,
les limit es des cernes ont pu être appréciées au
microscope à un fort grossissement (x 40). Elles sont
caractérisées par une alternance de bande de parenchymes. Les
deux zones d'études ont été enfin reconnues comme des
milieux subissant de fortes pressions anthropi ques par une utilisation
régulière et fréquente des feux de saison sèche.
Ainsi l'utilisation des pare -feux pour préserver les zones à
grand risque s'avère nécessaire.
Mots clés: Datation, Dendrochronologie,
Anatomie du bois, feu de végétation, savanes, Afrique de l'Ouest,
Monts Couffés (Bénin), Parc National de Comoé (Côte
d'Ivoire)
1. INTRODUCTION
1.1. Background on tree dating methods
Knowledge of the age of trees has a number of implications.
Firstly, tree dating, combined with knowledge on stand structure can give
information about forest disturbance. Therefore, it is often used to study the
forest dynamics (Mundo et al., 2007). It also prevents the loss of
genetic diversity and allows foresters to develop sustainable harvest pr
actices (Roel, 2005). The knowledge on age and increment growth of the trees
also sheds new light on global climate models (Gerhard et al., 2004;
Walter, 2004; Bouriaud et al., 2005; Schöngart et al.,
2006; Bütgen et al., 2007; Thomas, 2007). To estimate tree a
ge, scientists use two major methods. The first is the relative dating that
regroups the periodic annual increment (PAI) method and the crown class model
(Backer, 2003). These methods are not always accurate tree dating. They are
based on diameter growth without taking in consideration that many other
factors like soil fertility could influence tree-growth. Therefore, they can
overestimate or underestimate the age of trees.
To address this deficiency, dendrochronology also called
Tree-ring analysis was proposed in 1901 by Ellicott Douglas (1867-1962). He was
the first to remark that each year, trees add a layer of wood to its trunk and
branches and then producing annual rings. This approach has a lot of advantages
for forest management studies (Brienen , 2005). First, tree-ring
analysis gives information on the real age of a tree and the lifetime growth
rates and is therefore more effective than relative methods. It can be used to
reconstruct past disturbance (Brienen et al., 2007; Patrick et
al., 2008). For example, tree-rings are often used to reconstruct fire
history from fire scars (Welsberg & swanson, 2001; Guyette &
Stambaught, 2004; Van Horne & Fule, 2006; Hall, 2008). Another advantage of
the ring analysis is the possibility it offers to quantify variation in growth
among individuals over long periods of time (Desta et al., 2003).
Tree-ring analysis is also a good complementary tool to permanent sample plot
measurements. It needs only short time to provide many d ata on tree life.
Finally, tree-ring is used to reconstruct atmospheric gas concentration over
the past (Kennichi et al., 2004; XingYun et al., 2006;
Kristopher et al., 2007; Louise et al., 2008).
Despite all these advantages, this method has sometime s
limited applications in the tropics because of invisible rings in certain
species (Pascale et al., 2004). Also, it is only useful for trees
that are less than 600 years old (Worbes, 2002). However, it has been
demonstrated in
recent reports that some trees live more than 1000 years (Miguel
& Elena, 1998; Patrut et al., 2007).
Therefore, an alternative absolute dating method like
Radiocarbon is necessary. This approach was developed by Willard in 1946. The
radiocarbon method is more effective than all other approaches (Ramsey, 2007).
The dating method was used to explain some ecological and paleoclimatological
phenomena (Anouk et al., 2004; Patrut et al., 2007). But its
application requires well equipped laboratory and thus, it is more expensive.
According to Worbes (2002), it is better to use dendrochronology when tr ees
show visible rings. Many recent studies have also reported the presence of
annual ring in tropical species that may allow the use of tree-ring analysis as
dating tool (Miguel & Elena, 1998; Worbes, 2002; Dezzeo et
al., 2003; Fichtler et al., 2006; Patrut et al.,
2007).
In the present study we established the growth performance
from sapling s to trees for five savanna species (Isoberlinia doka
Craib and Stapf, Pterocarpus erinaceus Poir., Anogeissus
leiocarpa (DC.) Guill. & Perr., Detarium microcarpum Guill
& Perr., and Diospyros abyssinica (Hiern) F. White) of
West Africa. All of these species are found in open natural stands that were
subject to periodical bush fire. The main research question in these savanna
stands is related to the time the trees species, especially the endangered ones
needs to become fire-resistant. All of the five targeted species showed visible
annual tree -rings (Nigärd et al., 2004; Tarhule &
Leavitt, 2004; Poussart et al., 2006; Shöngart et al.,
2006) and allowed the use of tree-ring analysis for the study.
1.2. Interest of the study
Savanna ecosystems of West Africa including those of Benin and
Côte d'Ivoire are overexploited. Agriculture by burning, village hunting,
overgrazing and fire misuse increased the fragmentation of species habitats
with increasing shortage of biodiversity and climate change as direct feedback.
Fire misuse profits just within short time only for its users but its
disadvantages span over a longer time for all mankind. This threat on sudanian
resouces lead to the disappearance of plant and animal sp ecies. The
conservation of the endangered species should require a great description and
analysis of their habitats. For that purpose, research on the population
dynamics of tree-species in their ecological biotop e become important to
understand destructive process of anthropogenic pressure on the habitats of
species. Tree species like A. leiocarpa, I. doka, P. erinaceus, D.
microcarpum, and D. abyssinica are characteristics of the savanna
areas from Upper Aguima C atchment (Benin) and Comoé
National Park (Côte d'Ivoire) and some of these species
are endemic to the sudanian region depending the phytogeographical
classification of White (1983). Therefore, this research aimed to trace the
fire history from fire scars o bserved on the tree-rings. Thus, the frequency
of fire use in these studied areas and the number of years required for these
five species to become fire-resistant will be determined.
1.3. Aims
The study aimed at contributing to a better management and
conservation of West African tree species on the basis of their annual growth.
Therefore, five specific objectives were defined:
> to describe the wood anatomy of the sampled tree species;
> to determine the age of sampled trees;
> to determine the growth performances of sampled species in
their biotope ; > to synchronize fire scars for each of these species;
> to analyse fire regime in both sites.
1.4. Research questions
To achieve the above objectives, the following questions were
raised:
> does wood anatomy vary among species ?
? can the observed tree rings be interpreted as annual growth
?
> how long do tree species need to reach the height of 1.3 m
chosen as reference for dbh measure?
> which one of the studied site experienced more treated by
fire year round events?
2. STUDY AREA
The working material (sample tree discs) came from the
Comoé National Park (CNP, Côte d'Ivoire) and the Upper Aguima
Catchment (UAC, Benin). The CNP is situated between 8° 41'- 8° 44' N
and 3° 47'- 3° 51' W. The UAC is located between 9° 12'-
9°15' N and 1° 90- 1° 92 E.
Comoé National Park is comprised in interfluvial
peniplain of schist and granite with a mean altitude of 250 m to 300 m. The
geological subsurface of Upper Aguima Catchment is granite or gneiss with
typical ferralitic soils (Orthmann, 2005).
The two sites are characterized by alternating rainy and dry
season with mean annual rainfall and temperature of about 1150 mm and
26.5°C - 27°C respectively (Orthmann, 2005; Hennenberg, 2005). Dry
period occurs from November to February (Figure 1). The CNP is in the
borderline between the centre and the Guinea -Congolian / Sudanian transition
region (White, 1983 in Schöngart 2006). Its annual rainfall varies from
856 to 1248 mm (F ischer et al., 2002 in Hennenberg, 2005) and the
temperature fluctuates from 10°C to 40°C following seasons
(Hennenberg, 2005; Schöngart, 2006). The other one site is in Sudanian
regional centre of endemism (White, 1983). Its temperature ranged less than
15°C to more than 40°C among periods. Figure 1 shows the rain fall
trend per month for the studied areas.
250 200
|
|
150
|
|
100
^ainf
D .
D ( D C
|
|
50
|
|
0
|
|
|
J
|
F
|
M
|
A
|
M
|
J
|
J
|
A
|
S
|
O
|
N
|
D
|
CNP
|
5
|
11
|
40
|
82
|
132
|
170
|
190
|
212
|
207
|
88
|
9
|
9
|
UAC
|
9
|
28
|
65
|
120,5
|
130
|
128
|
100
|
140
|
205
|
121
|
30
|
10
|
Months
Figure 1: Mean monthly rainfall for studied
sites (CNP and UAC). Period from 1960 to 2001
The dry season of about four months is more remarked in
Comoé National Park than Upper Aguima Catchment. However, during the
rainy season less precipitation can be observed at UAC from May to September
than in the CNP (Fig. 1). This could influence differently the plant species
growth.
The Upper Aguima Catchment site is located in Sudanian
regional centre of endemism following White's phytogeographical classification
of Africa. The vegetation of the site is therefore mapped as savanna and open
forest. It is characterized as undifferentiate d woodland that included trees
with an understory of grasses, shrubs and herbs. The trees are mainly deciduous
in the dry season. Typical tree species are A. leiocarpa, Acacia
seyal, Kigelia africana and species of Combretum and
Terminalia genus. In this ecoregion, the dominance of I. doka
was also noted (White, 1983). As far as the Comoé National Park is
concerned, it is classified in IUCN category II and located in two different
phytogeographical regions. The south-western part of the park is in the
Sudanian zone while the northern part is in Guineo -
Congolian/Sudanian regional transition zone. The vegetation of
this transition zone is described as mosaic of dry, peripheral, semi -evergreen
rainforest and woodland and secondary grassland. Thus, it is noted the presence
of some savanna tree species like D. microcarpum, A. leiocarpa, Daniellia
oliveri, I. doka, P. erinaceus etc...
3. MATERIAL AND METHODS
3.1 Studied plant species and collection of samples
This research aimed to establish the growth performance for
five tree species from four different wood species families (Table 1). The
Samples were collected in 2002 in the Comoé National Park (Côte
d'Ivoire, Hennenberg, 2005) and in Central Benin (Orthmann, 2005). At CNP, 10,
8 and 8 samples were respectively collected from D. microcarpum,
A. leiocarpa and D. abyssinica. A. leiocarpa was
collected at the forest border, D. microcarpum in the savanna and
D. abyssinica in the forest. In Benin, six (6) samples were also
collected from I. doka, P. erinaceus and A. leiocarpa
in open mosaic forest.
TABLE 1- The five tree species investigated
in two different sites. UAC: Upper Aguima catchment, CNP: Comoé National
Park; Cutting levels (level 1: cut at 0,1m - level 2: cut at 1,3m) and N (tree)
means the number of sampled trees.
Site
|
Species
|
Family
|
Foliation pattern
|
N (tree)
|
Cutting levels
|
CNP
|
Anogeissus leiocarpa (DC.) Guill. & Perr.
|
Combretaceae
|
Short deciduous
|
8
|
1-2
|
UAC
|
Anogeissus leiocarpa (DC.) Guill. & Perr.
|
Combretaceae
|
Short deciduous
|
6
|
1
|
CNP
|
Detarium microcarpum Guill & Perr.
|
Caesalpiniaceae
|
Deciduous
|
10
|
1-2
|
CNP
|
Diospyros abyssinica (Hiern) F. White
|
Ebenaceae
|
Evergreen
|
8
|
1-2
|
UAC
|
Isoberlinia doka Craib and Stapf
|
Caesalpiniaceae
|
Brevi-deciduous
|
6
|
1-2
|
UAC
|
Pterocarpus erinaceus Poir.
|
Fabaceae
|
Pronounced deciduous
|
6
|
1
|
The african birch species named Anogeissus leiocarpa
(DC.) Guill. & Perr., belong to the family of Combretaceae and ranged
from the borders of the sahara up to the outlier humid tropical forests and is
found from Senegal to Cameroon in West Africa. The tree of about 20 m of height
with light green foliage grows in dry forest s, fringing forests and semi aris
savanna areas. The leaves, bark, roots and seeds serve for traditional tanning
and its wood is used for house construction and tool handles because of its
resistance against insects (Seed leaflet, 2007). The picture 1 shows some of
morphological characteristics of the species.
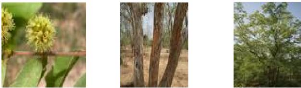
1a 1b 1c
Picture 1: Morphological characteristics of
Anogeissus leiocarpa
Picture 1a: Flowers - Picture 1b: aspect of the stem - Picture
1c: the tree (Brunken et al., 2008)
Detarium microcarpum Guill. & Perr. from
the family of Caesalpiniaceae typically found in high rainfall savanna areas,
dry forests and fallow land, on sandy or iron rich hard soils. It can grow up
from 10 m to 25 m in height. D. Microcarpum tree is also found in open
savanna as a more stunded tree with smaller fruits as showing the picture 2. It
has great fruit-bearing potential and is also used as medicinal plant.
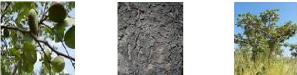
2a 2b 2c
Picture 2: Morphological characteristics of
Detarium microcarpum
Picture 2a: the fruits - Picture 2b: the aspect of the bark -
Picture 2c: the tree (Brunken et al., 2008)
Diospyros abyssinica (Hiern) F. White has small,
medium or large tree up to 36 m with a relatively sparse and shortly
branched crown. The leaves are altern ate, glossy dark green and distinctly
red when they are young. Its ecological distribution is known as widespread
in
tropical Africa from the Guinea Republic to Eritrea southwards
to the FZ area an d Angola. It is known for its medicinal use and used to make
pestles. The picture 3 shows at left the leaves and at right its trees in
forest stand.
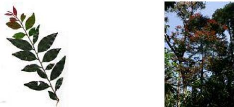
3a 3b
Picture 3: Morphological characteristics of
Diospyros abyssinica Picture 3a: a leave - Picture 3b: the tree
(Brunken et al., 2008)
Isoberlinia doka Craib Stapf is one of several
sudanian species of the family of Caesalpiniaceae found in deciduous woodland,
tall grass savanna alway s in stands forming open forests with Isoberlinia
tomentosa, Burkea africana, Prosopis africana, Uapaca somon etc... The
tree has 10 to 20 m of height and the trunk straight 5 m height. The wood is
used in marine construction, naval architecture, in paper products, as pulpwood
and for office materials. The aspect of the foliage is presented in figure
4.
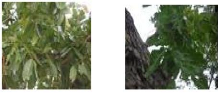
4a 4b
Picture 4: Morphological characteristics of
Isoberlinia doka
Picture 4a: the fruits - Picture 4b: the aspect of the bark
(Brunken et al., 2008)
Pterocarpus erinaceus is a tropical African deciduous
tree of 12 to 15 m (or more) that can be found in open stands, woodland and
savanna. The bark is fissured as showing the picture 5.
Its wood is useful for house construction, in carpentry for doors
and windows frames. It makes very good charchoal and decorative things.
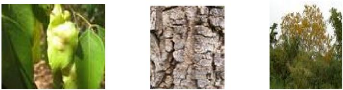
5a 5b 5c
Picture 5: Morphological characteristics of
Pterocarpus erinaceus
Picture 5a: the fruits - Picture 5b: the aspect of the bark -
Picture 5c: the tree (Brunken et al., 2008)
The dendrometric measurements such as diameter at 10 cm from
grou nd, diameter at breast height (DBH) and the total height were made on some
of the sampled trees by Orthmann (2005) and Hennenberg (2005). Their mean
values were presented in table 2. The cross-sectional wood discs were cutted at
10 cm from ground level and at breast height (DBH) for all tree samples from
Ivory Coast. In Benin, at least one stem disc per tree was cutted.
TABLE 2- Some characteristics of the
investigated species. MD (1): mean diameter at 10 cm from ground, MD (2): mean
DBH, MNR: mean number of ring of samples cutted at 10 cm from ground, MH: mean
height and s.d: standard of deviation.
Species
|
N (trees)
|
MD (1) #177; sd (mm)
|
MD (2) #177; sd (mm)
|
MNR
|
MH #177; sd (m)
|
A. leiocarpa (UAC)
|
6
|
44 #177; 1.41
|
|
6.33 #177; 0.82
|
1.8 #177; 0.00
|
A. leiocarpa (CNP)
|
8
|
72.62 #177; 4.14
|
52.12 #177; 1.81
|
17 #177; 1.41
|
6.77 #177; 0.52
|
D. microcarpum
|
10
|
79.5 #177; 10.01
|
52.1 #177; 1.29
|
13.4 #177; 2.12
|
3.47 #177; 0.32
|
D. abyssinica
|
8
|
76.62 #177; 12.45
|
52.75 #177; 2.12
|
30.25 #177; 2.55
|
7.00 #177; 0.71
|
I. doka
|
6
|
55.25 #177; 7.91
|
42.44 #177; 3.50
|
7.83 #177; 2.40
|
2.77 #177; 0.55
|
P. erinaceus
|
5
|
42.93 #177; 2.96
|
|
8.2 #177; 1.09
|
2.14 #177; 0.22
|
The stem discs were labelled and conducted in laboratory
(University of Göttingen, Germany). It is useful to note that the most of
Comoé National Park samples were really cutted at two levels of height
(10 cm from ground and 1.3 m). The missing diameter values were added after
measurements on these stem discs with electronic forest compass (Pictures 6 and
7).
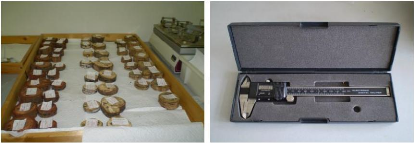
Picture 6: Some of the analysed stem discs
Picture 7: The used electronic forest compass
3.2 Sample processing
The stem discs were polished successively with different bands
using a polishing machine (Picture 8). The band of 80 um was first used and
followed by grains of 120, 240, 400 and 600 um. After this step, the wood dust
was cleaned from sample surface with electrical air pump. These applications
helped us to make good macroscopic observation s of the rings boundaries
(Figure 1). The different rings of each disc sample were then marked using a
pencil. A magnifying glass was sometimes used to identify the ring when the
macroscopic observation was not easy.
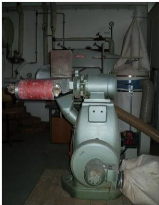
Picture 8: The used polishing machine
3.3 Method used to study the wood anatomy
Before describing the wood structure, we defined some criteria
that were mostly based on wood structure following cell types: vessel elements
and its distribution, disposition of rays and parenchyma cells. For that, we
referred to Coster (1927, 1928), Worbes (2002) and Shöngart (2006).
Following these criteria, the macroscopic and microscopic observations were
done using a scanner and the Leica software with magn ifying glass and
microscope.
3.4 Method used to study the growth performance s
The growth performances were mostly based on the age of trees,
ring increment and the initial growing in height. They helped to assess the
differences between the species. To determine the age of each sampled tree, the
rings were easily counted visually. However, all measurements (tree ring
counting and ring width measurement) were done with high accuracy using
electronic microscope and Lintab (Picture 9). The number of rings identified on
wood discs cutted at 10 cm from ground indicates after a cross dating, the age
of specified tree. The number of years needed by the targeted trees to reach
the breast height (1.3 m) was determined making a difference between the
numbers of observed rings on two stems discs (the stem disc cutted at 10 cm
from ground and the one cutted at 1.3 m) from a same tree completed by cross
dating.
Tree-rings widths were measured using the software TSAPWin by
digital measuring device Lintab 5, Factor: 1, 00 and using 1/100 mm as Length
unit, COM port 4 and PC Mouse. To compare the variation in growth performances
between species, the data on the ages and mean annual ring increment (MRI) were
analysed with statistical approach. The test of ANOVA followed by
Student-Newman-Keuls was used after a logarithmic transformation to stabilize
the variance and normalize the data.
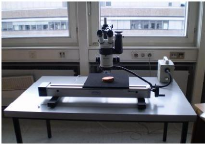
Picture 9: The Lintab combined to microscope for
measurement
3.5 Cross-dating
Cross dating of time series was used for the verification of the
series and the elimination of possible errors and to find the correct dated
position in time.
It helped in the elimination of measurement errors, e.g. the
removal of «false rings» and the insertion of «missing
rings». TSAP-Win offered a combination of both visual (graphical)
and statistical cross-dating. Statistical models are excellent tools to find
possible matches or to verify the dates of pre-dated time series.
In dendrochronology two main concepts are used to express the
quality of accordance between time series: Gleichlaeufigkeit and/or t -values.
While the t-statistic is a widely known test for mean difference significance,
Gleichlaeufigkeit was developed as a special tool for cross-dating of tree-ring
series (Eckstein & Bauch, 1969). These concepts are characterized
by a different sensitivity to tree-ring patterns. While Gleichlaeufigkeit
represents the overall accordance of two series, t-values are sensitive to
extreme values, such as event years. A combination of both is realized in the
Cross-Dating Index (CDI) that was used in this study
to obtain the exact year in witch each tree ring was formed
and also for matching variation in ring width among several tree rings series.
To identify real age of each tree, the cross -dating was done between the two
series time of the stem disc cut at 10 cm from ground and the other one felt at
1.3 m of height. However, for samples cutted only in one level of tree (level
1), it was done between different trees of the same species and from the same
stand.
3.6 Method used to analyse fire regime
According to Multilingual Glossary of Dendrochronology, fire
regime means the combination of fire frequency, intensity, size and seasonality
that determine the role of fire in a given ecosystem. When a bush fire passes,
the trees of this specified site are injured forming the fire scares that are
often observed on cross wood section. In the present study, these fire scares
were used to assess the fire interval, its frequency and intensity for
comparing both studied sites.
Fire interval means the number of years between two consecutive
fi res scars in a specific cross-wood section.
Fire frequency differs from fire interval in the sense that it
means the number of fires per year in a given area. To determine this, we
examine d fire season by their intra annual position in the fire scars using
high accuracy. The scars observed in ring boundary were formed during dry
season and those that were observed in ring growth zone occurred during the
growth season (rain period).
To assess the intensity of each fire, all the stem discs were
examined. For individual tree, a fire is qualified as low intensity when the
scar was observed only in sample cut ted at 10 cm from ground. When the same
fire was identified from both samples of height (10 cm and 1.3 m) for a same
tree, it was considered as a high intensity fire. However, we carefully
completed this analysis with the number of tree that recorded the fire and
sometimes we analyzed the season of fire that allowed confirming the
observations. We must precise that most discs samples were cutted from two
levels in Comoé National Park that is not the case in Upper Aguima
Catchment where the most stem discs were only collected at 10 cm from
ground.
3.7 Nature of data
Quantitative and qualitative data were obtained from the stem
discs analysis. The qualitative data were obtained through macroscopically
and micr oscopic appreciation of the wood
colour, the analysis of rings structure, the distribution of
vessels and parenchyma that helped to describe the wood anatomy of the species.
The position (year) of the identified fire scares on cross wood was also a
qualitative data that allowed the determination of the years of bush fire from
sampled trees.
The quantitative data were the number of tree rings per stem
discs, their widths, the number of scarred trees per species and the number of
rings scarred on each stem disc. To do this, it was necessary to prepare the
stem discs by their processing.
4. RESULTS
4.1 Wood anatomy of the species
All dendrochronological investigations of the study were based
on the hypothesis that wood structure varies considerably following the
species. The Figure 2 shows the difference between the species in wood colour,
the aspect of the bark and the distinctiveness of the rings boundaries
depending of the cross-wood sections which were used for analyses.
Specifically, the figure 3 and 4 were chosen for the description of the common
legend of the wood anatomically.
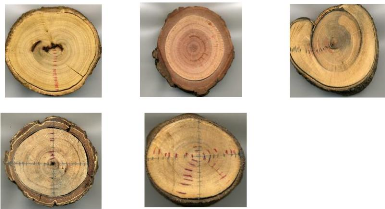
a b c
d e
Figure 2: Macroscopically polished stem discs
observation of investigated species. Fig a. - Fig
b. D. microcarpum (Caesalpiniaceae) - Fig c. D.
abyssinica (Ebenaceae) - Fig d. I. doka (Caesalpinaceae) - Fig e.
P. erinaceus (Fabaceae).
Inner bark
Pith
Outer bark Outermost ring
|
|
Heartwood Sapwood
Cambium
Innermost ring
|
Figure 3: The different parts of wood. Stem
disc of D. microcarpum
Ring boundaries
(marginal parenchyma band)
|
|
Vessels
Radius
Parenchyma
|
|
|
|
Figure 4: The common wood anatomical
4.1.1. Anogeissus leiocarpa
The bark of A. leiocarpa is slightly furrowed with
dirty white drawing sometimes ashy in colour. The sapwood is yellowish and the
difference bet ween heartwood is not so remarkable in young tree.
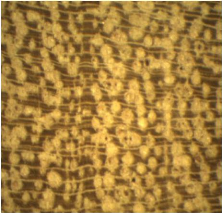
Figure 5: Wood anatomy of A. leiocarpa
(Combretaceae)
From pith to cambium, we observed the thin radiu ses which are
very near one another. The growth type zone shows variations in the vessel
distributio n (Figure 5). The border of ring is presented like single circular
line. For the samples of UAC (Benin), it was not always easy to identify
macroscopically the rings boundaries when they are narrows. But, all the CNP
samples showed distinct tree-rings by visual observation. Only in stem discs
from CNP, an alternating of early wood and latewood was identified. The
latewood s are very hard and darker. The distinctiveness of ring boundary in
A. leiocarpa trees depends also on the ring size and the environmental
conditions. A. leiocarpa, samples collected from two different sites
showed variation in ring visibility. The samples from CNP have best distinct
rings that may be explained by month rainfall diagram. In this area, the dry
season occurs from November to February like in Benin site (UAC) but it is more
marked. Thus, A. leiocarpa has high tree-ring sensitivity. It is
strongly influenced by changes in moisture conditions. A. leiocarpa
trees react strongly to environmental factors.
4.1.2. Detarium microcarpum
The outer bark was moderated furrowed and greyish with reddish
inner bark. The wood of D. microcarpum is hard and dark brown in
colour. The sapwood was lighter than heartwood. The polished stem disc showed
good distinct tree-rings (Figure 6).
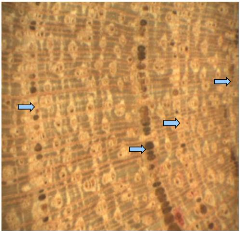
Figure 6: Wood anatomy of D. microcarpum
(Caesalpiniaceae)
There are many parallel rays mostly in sapwood which are
perpendicular to tree rings. In these rays, we identified radial parenchyma
cell s. The small vessels are distributed in all sapwood. No vessel has been
noticed in heartwood. The pith is characterized by spongy tissue. Tree-rings
are darker in colour and were formed by consecutive vessels in single line for
heartwood. The vessels of rings boundaries are bigger. Two successive vessels
are separated by one radius. The radiuses are wide and the growth border was
characterized by marginal parenchyma bands. In sapwood, we did observe that the
growth ring boundary was delimited by single concentric line. The number of
vessels in growth ring border decreases from heartwood to sapwood. Sometimes,
no vessel was noticed in concentric line.
4.1.3. Diospyros abyssinica
The bark of D. abyssinica is black spotted white in
colour. The sapwood is slightly different from heartwood in colour.
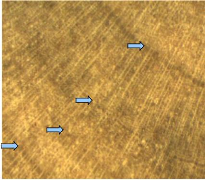
Figure 7: Wood anatomy of D. abyssinica
(Ebenaceae)
The wood colour is usually between white an d yellow and the
heartwood presents black streaks (Figure 7). The identification of tree-rings
is not always easy macroscopically. They are narrow and are presented like
single concentric line. In the growth zones, no vessels were observed. The
boundary zones are characterized by patterns of alternating parenchyma and
fibre bands.
4.1.4. Isoberlinia doka
I. doka tree has moderately furrowed bark whose
colour varies from white to brown. In the studied samples, there were no
significant colour differences between heartwood and sapwood. The wood is
usually brown (Figure 8).
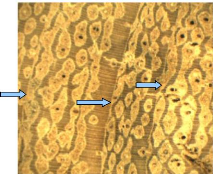
Figure 8: Wood anatomy of I. doka
(Caesalpinaceae)
Tree-rings are darker and wide. They appear like concentric
bands. Thus, macroscopically the boundary zones are distinct and are formed by
tangential lines within a tree ring. In wood cross section, vessels of fairly
uniform are distributed throug hout a growth ring (diffuse-porous). Therefore,
Tree-rings are characterized by marginal parenchyma bands. All vessels are
housed in storage cells (radial parenchyma) that are lighter and show a
parallel disposition to the boundary zone. In each radial par enchyma, we
identified one to three vessels. The radial parenchyma was mostly seen in
sapwood. The Figure 8 shows visible annual ring in I. doka wood stem
disc. In the central core of this stem we noticed a small hole that
demonstrates the unlignified cell walls of pith.
4.1.5. Pterocarpus erinaceus
P. erinaceus bark is deeply furrowed with dark mahogany
in colour. The wood is yellowish and no difference was noticed between sapwood
and heartwood (Figure 9).
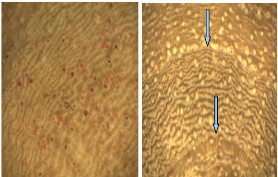
Figure 9: Wood anatomy of P. erinaceus
(Fabaceae).
The observed structure through high accuracy showed a
decreasing of vessels size towards the tree-ring. Then, just after one ring,
the vessel is wide and the size decrease s gradually until the next boundary
growth, thus a clear distinction of the rings. The boundary growth has circular
slightly undulating form. The presence of alternating fibres and parenchyma
tissues is remarkable.
4.1.6. Conclusion on wood anatomy
For each investigated species, the wood anatomical structure
showed a variati on from one to another. We also identified three different
tree-ring structures:
> border of rings presenting variation in vessels distr
ibution that was the case of A. leiocarpa (Combretaceae) and P.
erinaceus (Fabaceae) species;
> border of rings delimited by marginal parenchyma bands which
was represented by D. microcarpum (Caesalpiniaceae) and I. doka
(Caesalpiniaceae) species;
> growth ring boundary like alternating bands of fibre and
parenchyma cells illustrated by D. abyssinica (Ebenaceae).
The visual analysis demonstrated that there are differences in
tree-ring structures among the species. Thus, genetic impact is
questionable.
On the other hand, throughout the wood colour, we categorized the
targeted species in three major groups:
> white to yellow with black streaks wood illustrated by
D. abyssinica;
> yellowish wood that regroups A. leiocarpa and
P. erinaceus;
> light brown to dark brown wood that are the case of D.
microcarpum and I. doka. Both species are in the same family of
Caesalpiniaceae .
Finally, about the distinctiveness of ring borders, we
conclude that I. doka, D. microcarpum, and P. erinaceus
have the best distinct rings. As far as the D. abyssinica is
concerned, it showed annual tree-rings but the use of high accuracy method is
necessary.
4.2. Age and growth performances for investigated
species
4.2.1. Age, ring increment and cross-dating
The results of the cross dating process are summarized in
table 3 and show ed a great difference between the species depending of the
genetics characteristics and the growth conditions of the a tree.
TABLE 3 - Age and MRI of the investigated
species. MRI= Mean Ring Increment values. SNK = Student - Newman - Keuls
grouping of the species according to age and MRI (logarithmic transformation
was applied before the ANOVA test). Values with the same letter are not
significantly different. m= mean; s= standard deviation and do: error margin
for 95% of probability.
Species
|
Age (years)
|
MRI (mm)
|
m
|
s
|
do
|
SNK grouping
|
m
|
s
|
do
|
SNK grouping
|
D. abyssinica
|
30.25
|
2.55
|
1.77
|
A
|
1.19
|
0.22
|
0,15
|
D
|
A. leiocarpa (CNP)
|
17.00
|
1.41
|
0.98
|
B
|
1.90
|
0.61
|
0,42
|
C
|
D. microcarpum
|
13.40
|
2.12
|
1.31
|
C
|
2.02
|
0.31
|
0,19
|
C
|
P. erinaceus
|
8.20
|
1.09
|
0.95
|
D
|
2.32
|
0.29
|
0,25
|
C
|
I. doka
|
7.83
|
2.40
|
1.92
|
D
|
2.83
|
0.48
|
0,38
|
B
|
A. leiocarpa (UAC)
|
6.33
|
0.82
|
0.66
|
E
|
3.37
|
0.52
|
0,42
|
A
|
The youngest sampled species was A. leiocarpa from
UAC followed by the group of I. doka and P. erinaceus and the
oldest was D. abyssinica. The annual radial growth is great for young
trees and low for the oldest. These findings proved that the age and the mean
annual ring increment varied from one species to another, between trees and
also from one stand to another. According to these results, A. leiocarpa
trees from UAC, the youngest trees, showed the best mean annual radial
growth that was twice more than those of CNP. In fact, the targeted trees of
UAC (Benin) were between 5 to 7 years old and all had 1.8 m of height. The
diameters at 10 cm from ground vary between 4 cm and 5 cm. Those of CNP were 16
to 19 years old in 2001. Their height varied between 5.6 m to 7.1 m and the
diameters varied between 6.5 and 7.5 cm. D. abyssinica, the oldest
investigated trees showed the lowest radial growth witch is a characteristic of
the species. This variation of annual growth within the same tree is observed
in figure 10.
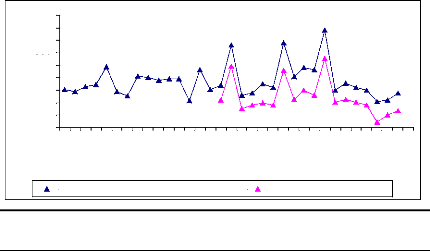
Figure 10: Cross-dating ring width of D.
abyssinica oldest sampled tree cut at two different heights. The yellow
curve was obtained from sample cut at 10 cm from ground and the ot her one was
for sample cut at 1.3 m from ground. Axe X describes the year and axe Y
presents the widths of rings
450
Time (Year)
1969
1979 1981
1997
1989
1977
1991
1971
1999
1995
1993
1987
1985
1983
1975
1973
2001
Rings width (1/100 m
400
350
300
250
200
150
100
50
0
Stem disc cutted at 10 cm from ground Stem disc cutted at DBH
The information about the lifetime of the oldest sampled tree
is present in figure 2. This figure was obtained using microscopic, TSAPWin
software in combination with lintab. The raw ri ng widths were cross-dated.
This curve shows the variation in annual ring increment from one year to
another. The tree germinated in 1969 and formed its first ring in 1970. The
highest ring width was observed in 1982 and the lowest was noticed in 1973 that
would probably be due to rainfall effect on tree growth in tropical area. T he
tree reached the height of 1.3 m in 1984 and proved the low growth rate of
D. abyssinica trees. The cross-dating index (CDI) is 23.5 (CDI >
10). This shows that the test of cros s-dating is very significant with the
probability of 99 %. The t-value (25.1) is greater than 3 and demonstrates the
high similarity between the two curves. Then, the statistic test proved a
similar radi al rate growth from ground to 1.3 m in D. abyssinica
trees. The same remark was made for D. microcarpum
trees.
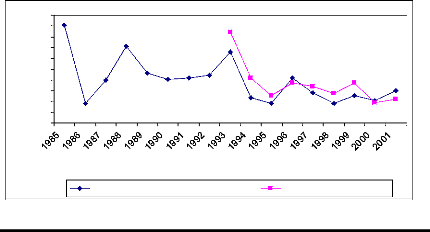
Time (Year)
500
Rings width (1/100 nn,
450
400
350
300
250
200
150
100
50
0
Stem disc cutted at 10 cm from ground Stem disc cutted at DBH
Figure 11: Cross-dating ring width of
Detarium microcarpum oldest sampled tree cut at two different
heights. The yellow curve was obtained from sample cut at 10 cm from ground and
the white curve was for sample cut at 1,3 m from ground. X describes the year
and axe Y presents the widths of rings
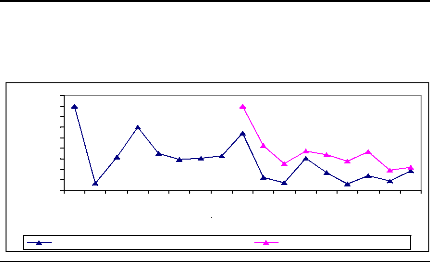
The oldest sample tree of D. microcarpum germinated
in 1984. It reached the DBH after eight years of life. The greater ring
increment was observed during the first year. The cross-dating test is
significant. The similarity between the two curves is also high.
4 5 0
4 0 0
3 5 0
3 0 0
2 5 0
2 0 0
1 5 0
1 0 0
5 0
0
Rings width (1/1( r,
1985
1986
1987
1988
1989
1990
1991
1992
1993
1994
1995
1996
1997
1998
1999
2000
2001
Time (Year)
Stem disc c u tte d a t 1 0 c m from ground Stem disc c u tte d a
t D B H
Figure 12: Cross-dating ring width of one of
sampled tree of A. leiocarpa cut at two different
heights. The yellow curve was obtained from sample cut at 10 cm
from ground and the white curve was for sample cutted at 1.3 m of height. X
describes the year and Y presents the width of the rings.
This tree produced its first ring in 1985. It reached the height
of 1.3 m in 1992. The cross - dating index is significant and the similarity
between the two curves is high.
4.2.2. Growing in height
The height growth was used to estimate the number of years
needed by each species to be outside fire events. Table 2 presents the total
height of different investigated trees and the figure 13 informs about the
number of years needed by each species to reach the height of 1.3
m.
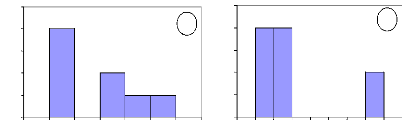
Number of individuals
4
2
5
3
0
1
a
Number of individuals
4
2
5
3
0
1
b
3 5 6 7
e of I Age
2 3 8
Age
by t
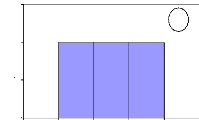
Number of individuals
3
2
0
1
c
3 6 7 9 15
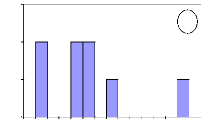
Number of individuals
2
3
0
1
d
3 4 5
Age
Age
Figure 13: Initial height growth for some of
investigated species. For all figures, the variable Age of abscise means
the number of years. (a) A. leiocarpa - (b): D. Microcarpum -
(c): I. doka - (d): P. erinaceus.
In these figures, axes X and Y describe respectively the
absolute frequency and the number of years. They show that the height growth
varies among species. Some savanna species such as A. leiocarpa, D.
microcarpum and I. doka need between 2 to 8 years to reach the
height of 1.3
m. However, D. abyssinica needs more time to reach the
same height of 1.3 m. In fact, this species grows slowly in wide and also in
height. It needs long time to be outside fire events.
4.2.3 Conclusion on tree species growth
A. leiocarpa, D. microcarpum and I. doka can
be categorized as fast-growing savanna species while D. abyssinica is
a slow-growing species. These observations are in accordance with those of
Worbes (1989). If we refer to anatomical characteriza tion described in our
results combined with the findings of Worbes (1989), we c ould assume a
relationship between wood colour and the growth performances. But, before
confirming this hypothesis, further studies on several savanna wood species are
necessary.
4.3. Fire regime analysis from tree -rings of
investigated species
4.3.1. Fire master chronology in CNP and UAC
The analysis of fire scars on investigated stem discs with
dendrochronology techniques helped to have the master fire chronology in both
sites as experienced by sampled tree that was presented in Figure 14 and 15.
Fire history in Comoe National Park
Number of scarred tree
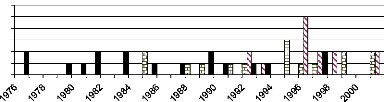
4
2
6
5
3
0
1
Forest border (A. leiocarpus, N = 8) Savanna (D. microcarpum, N =
10) Forest (D.abyssinica, N = 8)


Year
Figure 14: Fire history in Comoé National
Park as experienced by sampled trees. In Common
legend, N means the number of tree analyzed for each species.
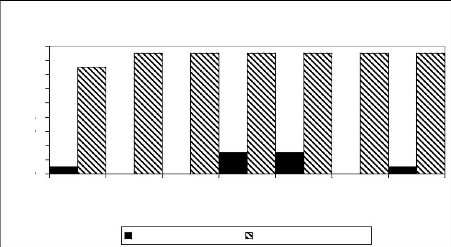
Number of scarred tree
18
16
14
12
10
4
2
8
6
0
1995 1996 1997 1998 1999 2000 2001
Fire history in Upper Aguima Catchment
Number of scarred tree Number of tree analyzed
Year
Figure 15: Fire history in Upper Aguima
Catchment as experienced by sampled trees.
4.3.2. Fire interval
In Comoé National Park, 19 different fire dates were
identified. The earliest fire of 1976 was recorded on two D. abyssinica
trees (D1-105 and D1-108). The first tree germinated in 1971 and has only
one fire scars and the other one germinated in 1974 with two different fire
scars. The recent fire in this park was recorded in 2001 on D. microcarpum
(DE-103; DE-105) and A. leiocarpa trees (AN-103; AN-105). The
highest number of fire scars was observed on D. abyssinica tree
(D1-104) that had five different fire scars (1981, 1985, 1987, 1990 and 1 992)
followed by one of A. leiocarpa tree (AN-104) which was scarred at
four different years (1988, 1991, 1995 and 1999).
Specifically, D. abyssinica trees that were collected
from forest area in CNP allowed us to date fire from 1976 to 1997. During this
p eriod, the fire interval varied from one to four years. Most of sampled wood
of D. abyssinica had their youngest fire scars before 1993. In 1997,
only two samples were scarred by fire. After this year, no fire scars was
observed on sampled cross wood of this species, indicating that fire
experienced by the sampled tree in forest of Comoé National Park was
restricted from 1997. This area was probably protected against fire by
technical forest management disposition.
About fire history in forest border, we estimated it from
A. leiocarpa samples from 1985 to 2001, the year before collecting
samples. It was estimated to be between four and six years until 2001. Thus, we
conclude that forest border of CNP was always treated by fire. A. leiocarpa
was described in CNP by Klaus (2006) as pioneer species. Thus we suppose
that the forest border was cleaned for fire breaks building. This observation
confirms the hypothesis that from 1997, forest area of CNP was protecte d
against fire. Its effectively was proven since 1997.
Concerning savanna area of this park, the fire past was
reconstructed from D. microcarpum species. All sampled trees for this
species were originated after 1985 and the earliest fire date in this area was
from 1992. From this year to 2001, the fire in terval varied between one and
five years. That could be a positive management action either for ensuring
grazing for herbivorous mammals or as to facilitate tourism. However, the
regular use of fire in CNP savanna area could also be due to the destructive
pressure of poachers during game searching.
In upper Aguima Catchment, the oldest tree collected from this
second studied area was twelve years old in 2002. The fire interval was from
1995 to 2001 with four different dates (1995, 1998, 1999 and 2001). On any of
samples, we identified more than one fire scar. However, like all samples were
collected in open mosaic forest, we can reconstruct fire history in this area
by dating the fire scar for each sa mple as shown in the figure 9. Thus, fire
interval in open mosaic forest of Upper Aguima Catchment was estimated between
one to three years. However, the all estimated fire interval depend of the
sampled tree that could escape fire event during the supposed -non fire period
even if bush fire occurs on year round cycle.
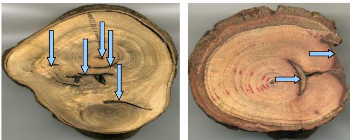
Figure 16: Example of different year's fire
scars on wood stem discs
4.3.3. Fire frequency and intensity in Comoé
National Park and Upper Aguima Catchment
In Upper Aguima Catchment, the fire frequency ranged from 1 to
3. In 1995, 1998 and 2001, the fire was used only during dry season but in
1998, three different fires were used consecutively (dry season, rain season
and at the end of rain season). In CNP, the fire frequency ranged from 1 to 2.
Most of them occurred during dry season when phytomass are abundant and dry.
The intensity of fire was assessed in CNP and five «high
fires date» were identified. These are originated from 1980, 1992, 1993,
1996 and 1997. The fire occurred during dry season when the combustible were
available.
4.3.4. Conclusion and discussion on events of fire in
savanna areas.
This study showed that the CNP needed more attention to become a
real biodiversity conservation area. The frequently use of fire caused a lost
of biodiversity.
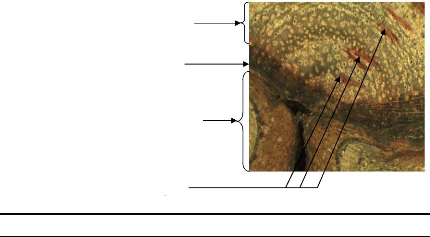
Compartment formed before bush fire scar
Fire scar
Compartment formed after bush fire scar
Annual rings
Figure 17: Illustration of compartmentalisation
concept on D. microcarpum stem disc
Concerning trees, when the stem was injured, the tree develops
a dynamic defence process which forms structural and chemical boundaries in
order to resist the spread of pathogens. In short time, the tree forms
compartment and this positive reaction of the tree is named
compartmentalisation. The wood formed just after a fire injury showed an
anatomical wood which is different from the normal. Most of time, no vessel was
observed in this part of wood but a callous margin (edge of callous tissue
overgrowing a stem wound) was formed.
5. DISCUSSIONS
Based on the findings of this study and following the wood
anatom ical classification of Worbes (1989), Fichtler (2003) and Schöngart
(2006) , the presence of annual tree-ring in tropical African species such as
A. leiocarpa, D. microcarpum, D. abyssinica, I. doka and P. erinaceus
is undoubtable. A dry period of at least two months with less than 50 mm
of rain would be required to expect annual rings in tropical tree-species
(Worbes, 1999). However, Fichtler et al. (2003) reported that even
small annual variation in rainfall occurring under everwet conditions may
trigger ring formation. This confirms the role of abiotic factor triggering on
tree growth such as the precipitation (Worbes, 1995; Fichtler et al.,
2004; Shöngart, 2006). Furthermore early studies and especially those
of Coster ( 1927) well illustrated the connection between the formation of
annual tree rings and seasonal precipitation. He found that trees of the same
species might form clear and annual rings under monsoon climates, while the
same species form distinct and irregular rings under almost everwet
conditions.
Concerning the growth performance, the results of Worbes
(1989) proved that whenever the pioneer species like A. leiocarpa grow
faster, those of the understory like D. abyssinica grow slowly and
constantly during all life time. He also demonstrated t hat within one species,
the mean annual growth can show a variation according to tree age. His findings
in 2003 proved also that the lowest values of diameter growth rates are
observed for understorey species whereas the highest values were noticed in the
main canopy and in emergent species. The young trees of A. leiocarpa
grew under good light conditions and showed therefore a good ring
increment. This high difference of growth within the same species could be
explained by the mean annual rainfall, index of the soil fertility and the
variation in disturbance following areas. However, the analysis of these
ecologic factors combined with the results about the ring increment of A.
leiocarpa from the two studied sites (Tables 2, 3 & 4) proved
that in this case, age of trees would be the main variable that caused the
variation observed. About D. microcarpum species, the trees grow fast
the first year with an annual ring increment of about 8 mm. The rate of growth
decreases the next years. In fact, the light is on e of most important limiting
factor on tree growth. According to Shöngart (2006), pioneer species are
highly light demanding for germination and growth, and are generally short
lived. The non -pioneer species have low light requirements and are able to
surv ive in the dark forest understorey, growing at low rates. The juvenile
trees of A. leiocarpa were found in gap dynamics (canopy
opening) and D. abyssinica trees were observed under
closed forest where less than 2 % of light penetrates.
According to Hennenberg et al. (2006), the use of
fires in Comoé National Park justifies a dramatic decrease of wild
animals. The results of his study proved that fire is more used in savanna area
than in closed forest. He demonstrated that the forest boundary was sometimes
treated by fire to about 30 m which was the consequence of using of wide forest
boundary of 60 m. This helps us to accept our hypothesis about closed forest
protecting against fire.
6. CONCLUSION
Dendrochronology was demonstrated in the present study like a
vital method of juvenile tree dating. All investigated species are sensible to
abiotic factors and especially rainfall that determine the distinctiveness of
ring boundary. The oldest sample tree was 33 years old whereas the youngest was
5 years old. The variability in wood anatomy of the studied species could be
used to understand the diversity in tropical forest. The events of fire on
tropical ecosystems and especially its impact on tree growth w as studied and
revealed that West African ecosystems are not always controlled by human
pressure. Further research works on these ecosystems with emphasis on
endangered species by using tree -ring analysis approach for developing news
forest management actions are need.
7. LITERATURE CITED
ADU-BREDU S., FOUA T. B. A., BOUILLET J. P., KOUAME M.
M., YAMOAH K. S., & SAINT-ANDRE L., 2008. An explicit
stem profile model for forked and un -forked teak (Tectona grandis)
trees in West Africa. Forest ecology and management, 255(7): 2189 -2203.
BAKER P. J., 2003. Tree age estimation for
the tropics: A test from the southern Appalachians. Ecological Applications
ISSN 1051-0761 CODEN ECAPE7; 13(6):1718- 1732.
BAKER P. J., PALMER G. J. & D'ARRIGO
R., 2008. The dendrochronology of Callitris intratropica
in northern Australia: annual ring structure, chronology development and
climate correlations. Australian Journal of Botany; 56(4):311-320.
BOURIAUD O., LEBAN J.-M., BERT D. &
DELEUZE C., 2005. Intra-annual variations in climate influence
growth and wood density of Norway spruce. Tree Physiology 25: 651-
660.
BRIENEN R. J.W., 2005. Tree
rings in the tropics: a study on growth and ages of Bolivian rain forest trees.
PhD Thesis, Universiteit Utrecht, 139p. PROMAB Scientific Series
10.
BRIENEN R. J.W., LEBRILA E., BREUGUEL V. M. &
MARTINEZ-RAMOS M., 2007. Dating tropical trees in secondary
forests along chronsequences. ESA/SER Joint Meeting. (10): 72-102.
BRUNKEN U., SCHMIDT M., DRESSLER S., JANSSEN T.,
THIOMBIANO A., & ZIZKA G., 2008. West African plants. A
photo Guide. w
ww.
Westafricanplants.senchenberg.de. -forschungsinstitut senchenberg,
Frankfurt / Main, Germany.
COPENHEAVER A. C., POKORSKI A. E., C URRIE J. E.
& ABRAMS D. M., 2006. Causation of false ring formation
in Pinus banksiana: A comparison of age, canopy class, climate and
growth rate. Forest Ecology and Management, 236
(2-3):348-355.
COSTER C, 1927. Zur Anatomie und Physiologie der
Zuwachszonen und Jahresringbildung in den Tropen I. Ann. Jard. Bot.
Buitenzorg, 37: 105-119.
CULLEN E. L., ADAMS A. M., ANDERSON J. M. &
GRIERSON F. P., 2008. Analyses of 13C and 18O in tree rings of
Callitris columellaris provide evidence of a change in stomatal
control of photosynthesis inresponse to regional changes in climate. Tree
Physiology 28: 1525-1533.
DESTA F. F., RAY R. H. J. & COLBERT
J., 2003. Influence of topographic aspect, precipitation and drought
on radial growth of four major tree species in an Appalachian watershed.
Forest Ecology and Management, 177(1-3):409-425.
DEZZEO N., WORBES M., ISHII I., & HERRERA
R., 2003. Annual tree rings revealed by radiocarbon dating in
seasonally flooded forest of the Mapire River, a tributary of the lower Orinoco
River, Venezuela. Vegetatio 168(1):165-175.
ENGLAND R. J. & ATTIWILL M. P.,
2007. Changes in sapwood permeability and anatomy with tree age and
height in the broad -leaved evergreen species Eucalyptus regnans. Tree
Physiology 27: 1113-1124.
FICHTLER E., CLARK A. D. & WORBES
M., 2006. Age and Long-term Growth of Trees in an Old-growth Tropical
Rain Forest, Based on Analyses of Tree Rings and 14C .
Biotropica, 35(3):306 - 317.
GUYETTE P. R. & STAMBAUGH C. M.,
2004. Post-oak fire scars as a function of diameter, growth and tree age.
Forest Ecology and Management, 198(1-3):183-192.
HALL L. R. M., 2008. The fire history of a
416-years-old western larch tree in southeastern Britisch Columbia. BC
Journal of Ecosystems and Management 9(2): 5 -10.
HENNENBERG K. J., 2005. Vegetation ecology of
forest -savanna ecotones in the comoe National Park (Ivory Coast): Border and
ecotone detection, core area analysis and ecotone dynamics. Ph-D
dissertation, University of Rostock (Germany). 108 pages.
HENNENBERG K. F., FISHER F., KOUADIO K., GOETZE D.,
ORTHMANN B., LINSENMAIR K. E., JELTSCH F. & POREMBSKI S.,
2006. Phytomass and fire occurrence along forest-savanna transects in
the Comoé National Park, Ivory Coast. Journal of Tropical Ecology
22: 303-311.
KENICHI Y., SACHIE I., TAKAYUKI K., EITARO F., YUTAKA
M., MITSUTOSHI K., HIROYUKI T., TAKAYOSHI K. & RYO F., 2004.
Effects of elevated CO2 concentration on growth, annual ring structure and
photosynthesis in Larix kaempferi seedlings. Tree Physiology 24:
941-949.
Martínez-Ramos M. & Alvarez-Buylla E.
R., 1998. How old are tropical rain forest trees? Trends in plant
science 3: 400-405.
MUNDO A. I, BARRERA D. M. & ROIG A. F.,
2007. Testing the utility of Nothofagus pumilio for dating a
snow avalanche in Tierra del Fuego, Argentina. Dendrochronologia,
25(1):19-28.
NYGÄRD R., SAWADOGO L. & ELFVING
B., 2004. Wood-fuel yields in short-rotation coppice growth in the
north Sudan savanna in Burkina Faso. Forest Ecology and Management, 18
(1-3):77-85.
NOVAK K., CHERUBINI P., SAURER M., FUHRER J., SKELLY
M. J., KRÄUCHI N. & SCHAUB M., 2007. Ozone air
pollution effects on tree -ring growth, 13C, visible foliar injury and leaf gas
exchange in three ozone -sensitive woody plant species. Tree Physiology 27:
941-949.
ORTHMANN B., 2005. Vegetation ecology of
woodland-savanna mosaic in central Benin (west Africa): ecosystem analysis with
a focus on the impact of selective logging. Ph-D dissertation, University
of Rostock (Germany). 137 pages.
PATRUT A., VON REDEN K. F., LOWY D. A., ALBERTS A. H.,
POHLMAN J. W., WITTMANN R., GERLACH D., XU L. & MITCHELL C. S.,
2007. Radiocarbon dating of a very large African baobab. Tree
Physiology 27:1569-1574.
POUSSART P. F., EVANS N. M. & SCHRAG P.
D., 2004. Resolving seasonality in tropical trees: multi-decade,
high-resolution oxygen and carbon isotope records from Indonesia and Thailand.
Earth and Planetary Science Letters, 218(3-4):301-316
POUSSAART P.M., MYNENI S.C.B. &
LANZIROTTI A. 2006. Tree-ring dating, age-dating trees by
counting annual rings. Geophysical Research Letters 3:L17711.
PRIYA P. B. & BHAT K. M., 1997.
False ring formation in teak (Tectona grandis L.f.) and the influence
of environmental factors. Wood Science Division, Kerala Forest
Research Institute Peechi, 680 653 India .
RAMSEY C. B, 2007. Radiocarbon dating:
Revolution in understanding. Archaeometry, 50(2):249 - 275.
SANTOSH K. S., AMALAVA B. & VANDANA
C., 2007. Reconstruction of June- September precipitation based on
tree -ring data of teak (Tectona grandis L.) from Hoshangabad, Madhya
Pradesh, India. Dendrochronologia, 25(1):57-64.
SAURER M., CHERUBINI P., BONANI G. &
SIEGWOLF R., 2003. Tracing carbon uptake from a natural CO2
spring into tree rings: an isotope approach. Tree Physiology 23:
997-1004.
SHÖNGART J., ORTHMANN B., HENNENBERG K. J.,
POREMBSKI S. & WORBES M., 2006. Climate-growth
relationships of tropical tree species in west Africa and their potential for
climate reconstruction. Global Change Biology, 12:1139-1150.
TARHULE A. & LEAVITT W. S., 2004.
Comparison of stable-carbon isotope composition in the growth rings of
Isorberlinia doka, Daniella oliveri, and Tamarindus indica and West
African climate. Dendrochronologia, 22(1):61-70.
THOMAS D. S., MONTAGU K. D. & CONROY J.
P., 2007. Temperature effects on wood anatomy, wood density,
photosynthesis and biomass partitioning of Eucalyptus grandis
seedlings. Tree Physiology 27:251-260.
BÜNTGEN U., FRANK D. C., KACZKA R. J., VERSTEGE
A., ZWIJACZ-KOZICA T. & ESPER J., 2007. Growth responses
to climate in a multi -species tree-ring network in the Western Carpathian
Tatra Mountains, Poland and Slo vakia. Tree Physiology 27:689-702.
VAN HORNE L. M. & FULE Z. P.,
2006. Comparing methods of reconstructing fire history using fire scars in a
southwestern United States Ponderosa pine forest. Can J. For. Res.36:
855-867.
VERHEYDEN A., KAIRO J. G., BEECKMAN H. &
KOEDAM N., 2004. Growth Rings, Growth Ring Formation and Age
Determination in the Mangrove Rhizophora mucronata. Annals of Botany, 94:
59-66.
www.aob.oupjournals.org.
VERHEYDEN A., ROGGEMAN M., BOUILLON S., ELSKENS M.,
BEECKMAN H. & KOEDAM N., 2005. Comparison between
ä13C of á-cellulose and bulk wood in the
mangrove tree Rhizophora mucronata: Implications for dendrochemistry.
ELSEVIER, Chemical Geology 219:275- 282.
WALTER O., 2004. Influence of climate on radial
growth of Pinus cembra within the alpine timberline ecotone. Tree
Physiology 24: 291-301.
WELSBERG J. P. & SWANSON J. F.,
2001. Fire dating from tree-rings in western cascades Douglas fire Forests: An
Error Analysis. Northwest Science, 75( 2).
WORBES M., 2002. One hundred years of tree-ring
research in the tropics - a brief history and an outlook to future challenges.
Dendrochronologia, 20 (1-2): 217-231.
WORBES M., STASCHEL R., ROLOFF A., & JUNK
W. J., 2003. Tree ring analysis reveals age structure, dynamics and
wood production of a natural forest stand in Cameroon. Forest ecology and
management, 173(1-3):105-123.
ZHAO X. Y., QIAN J. L., WANG J., HE Q. Y., WANG Z. L.
& CHEN C. Z., 2006. Using a Tree Ring
ä13C Annual Series to Reconstruct Atmospheric CO2 Concentration
over the Past 300 Years. Pedosphere, 16(3):371-379.
APPENDICES
Appendix 1: Some details on growth performances
of the species
Species
|
Nr of tree
|
Diameter (1)
|
Diameter (2)
|
Height (m)
|
Nr of rings (1)
|
Nr of rings (2)
|
MRI #177; s.d
|
A. leiocarpa (UAC) Combretaceae
|
1
|
42.2
|
|
1.8
|
5
|
|
4.03 #177; 2.04
|
2
|
43
|
|
1.8
|
7
|
|
3.66 #177; 1.69
|
3
|
47.05
|
|
1.8
|
6
|
|
3.63 #177; 1.79
|
4
|
41.2
|
|
1.8
|
7
|
|
2.82 #177; 0.99
|
5
|
45
|
|
1.8
|
6
|
|
3.40 #177; 2.77
|
6
|
43.11
|
|
1.8
|
7
|
|
2.70 #177; 1.24
|
A. leiocarpa (CNP) Combretaceae
|
1
|
75
|
50
|
6.5
|
19
|
14
|
1.05 #177; 0.57
|
2
|
65
|
50
|
6
|
16
|
13
|
2.04 #177; 1.13
|
3
|
76
|
52
|
6.3
|
16
|
10
|
3.22 #177; 1.92
|
4
|
72
|
52
|
7
|
19
|
16
|
1.70 #177; 0.67
|
5
|
76
|
55
|
7
|
16
|
13
|
1.86 #177; 0.87
|
6
|
77
|
51
|
6.5
|
16
|
12
|
2.00 #177; 1.29
|
7
|
70
|
53
|
7,1
|
18
|
15
|
1.69 #177; 0.67
|
8
|
70
|
54
|
5.6
|
16
|
14
|
1.65 #177; 0.67
|
D. microcarpum (Caesalpiniaceae)
|
1
|
89
|
53
|
3.5
|
12
|
9
|
1.67 #177; 0.85
|
2
|
90
|
54
|
3.8
|
13
|
9
|
2.60 #177; 1.34
|
3
|
69
|
51
|
3.5
|
12
|
10
|
2.12 #177; 1.18
|
4
|
73
|
50
|
3.2
|
11
|
8
|
1.85 #177; 1.79
|
5
|
81
|
51
|
4.1
|
16
|
12
|
1.93 #177; 1.77
|
6
|
62
|
51
|
3.3
|
13
|
11
|
1.67 #177; 0.90
|
7
|
92
|
53
|
3
|
17
|
9
|
2.28 #177; 1.09
|
8
|
80
|
53
|
3.2
|
12
|
9
|
2.33 #177; 1.49
|
9
|
73
|
52
|
3.6
|
12
|
9
|
1.85 #177; 1.19
|
10
|
86
|
53
|
3.5
|
16
|
8
|
1.89 #177; 0.83
|
D. abyssinica (Ebenaceae)
|
1
|
65
|
53
|
8
|
27
|
24
|
1.01 #177; 0.40
|
2
|
74
|
55
|
7.5
|
33
|
26
|
1.13 #177; 0.51
|
3
|
75
|
52
|
8.3
|
31
|
28
|
1.47 #177; 0.95
|
4
|
104
|
56
|
6.4
|
31
|
25
|
1.24 #177; 0.57
|
5
|
67
|
50
|
6.3
|
33
|
18
|
1.18 #177; 0.65
|
6
|
76
|
50
|
7
|
27
|
21
|
0.82 #177; 0.24
|
7
|
83
|
53
|
7.2
|
32
|
25
|
1.15 #177; 0.86
|
8
|
69
|
53
|
4.5
|
28
|
19
|
1.49 #177; 0.66
|
I. doka (Caesalpinaceae)
|
1
|
48.2
|
|
2.5
|
6
|
|
3.00 #177; 1.04
|
2
|
47.3
|
|
2.8
|
5
|
|
3.55 #177; 0.70
|
3
|
53
|
|
2
|
8
|
|
2.87 #177; 1.75
|
4
|
57
|
40.74
|
3.6
|
8
|
5
|
2.37 #177; 0.51
|
5
|
57
|
40.11
|
2.6
|
8
|
5
|
2.97 #177; 0.99
|
6
|
69
|
46.47
|
3.1
|
12
|
7
|
2.23 #177; 0.71
|
P.erinaceus (Fabaceae)
|
1
|
43.97
|
|
2.2
|
8
|
|
2.72 #177; 0.82
|
2
|
45.03
|
|
2
|
10
|
|
1.95 #177; 0.74
|
3
|
39.05
|
|
2
|
7
|
|
2.41 #177; 0.45
|
4
|
40.65
|
|
2
|
8
|
|
2.39 #177; 0.94
|
5
|
45.97
|
|
2.5
|
8
|
|
2.14 #177; 0.90
|
Appendix 2: The output of ANOVA test about the
chapter of growth performance
The SAS System 17:20 Friday, November
282008 2
The GLM Procedure
Dependent Variable: Age
Sum of
Source DF Squares Mean Square F Value Pr > F
Model 5 12.24798948 2.44959790 99.57 <.0001
Error 37 0.91028303 0.02460224
Corrected Total 42 13.15827251
R-Square Coeff var Root MSE Age Mean
0.930820 6.166173 0.156851 2.543734
The SAS System 10:23 Thursday, November 27, 2008 99
The GLM Procedure
Dependent Variable: MRI
Sum of
Source DF Squares Mean Square F Value Pr > F
Model 5 19.97423601 3.99484720 22.23 <.0001
Error 37 6.64786167 0.17967194
Corrected Total 42 26.62209767
R-Square Coeff Var Root MSE MRI Mean
0.750288 19.44599 0.423877 2.179767
Appendix 3: The output of Student-Newman-Keuls
test
Means with the same letter are not significantly different. Mean
= ln(Age)
SNK Grouping Mean N Sp
A 3.40633 8 4
B 2.83027 8 2
C 2.58458 10 3
D 2.09736 5 6
D
D 2.02074 6 5
E 1.83845 6 1
Means with the same letter are not significantly different. Mean
= ln(MRI)
SNK Grouping
|
Mean
|
N
|
Sp
|
A
|
3.3733
|
6
|
1
|
B
|
2.8317
|
6
|
5
|
C
|
2.3220
|
5
|
6
|
C
|
|
|
|
C
|
2.0190
|
10
|
3
|
C
|
|
|
|
C
|
1.9013
|
8
|
2
|
D
|
1.1863
|
8
|
4
|
Appendix 4: Fires dating from samples collected
in Comoé National Park
|
Trees
|
Level
|
76
|
79
|
80
|
81
|
83
|
85
|
87
|
88
|
89
|
90
|
91
|
92
|
93
|
95
|
96
|
97
|
98
|
99
|
2001
|
Tot
|
CNP
|
DE-101
|
0,1m
|
|
|
|
|
|
|
|
|
|
|
|
|
|
|
|
*
|
*
|
|
|
2
|
CNP
|
|
1,3
|
|
|
|
|
|
|
|
|
|
|
|
|
|
|
|
|
|
|
|
0
|
CNP
|
DE-102
|
0,1m
|
|
|
|
|
|
|
|
|
|
|
|
|
|
|
*
|
*
|
|
|
|
2
|
CNP
|
|
1,3
|
|
|
|
|
|
|
|
|
|
|
|
|
|
|
|
|
|
|
|
0
|
CNP
|
DE-103
|
0,1m
|
|
|
|
|
|
|
|
|
|
|
|
|
|
|
*
|
|
|
|
*
|
2
|
CNP
|
|
1,3
|
|
|
|
|
|
|
|
|
|
|
|
|
*
|
|
|
|
|
|
|
1
|
CNP
|
DE-104
|
0,1m
|
|
|
|
|
|
|
|
|
|
|
|
|
|
|
|
|
|
|
|
0
|
CNP
|
|
1,3
|
|
|
|
|
|
|
|
|
|
|
|
|
|
|
|
|
|
|
|
0
|
CNP
|
DE-105
|
0,1m
|
|
|
|
|
|
|
|
|
|
|
|
*
|
|
|
|
|
*
|
|
*
|
3
|
CNP
|
|
1,3
|
|
|
|
|
|
|
|
|
|
|
|
|
|
|
|
|
|
|
|
0
|
CNP
|
DE-106
|
0,1m
|
|
|
|
|
|
|
|
|
|
|
|
|
|
|
*
|
|
|
|
|
1
|
CNP
|
|
1,3
|
|
|
|
|
|
|
|
|
|
|
|
|
|
|
*
|
|
|
|
|
1
|
CNP
|
DE-107
|
0,1m
|
|
|
|
|
|
|
|
|
|
|
|
*
|
|
|
|
|
|
|
|
1
|
CNP
|
|
1,3
|
|
|
|
|
|
|
|
|
|
|
|
|
|
|
*
|
|
|
|
|
1
|
CNP
|
DE-108
|
0,1m
|
|
|
|
|
|
|
|
|
|
|
|
|
|
|
*
|
|
|
|
|
1
|
CNP
|
|
1,3
|
|
|
|
|
|
|
|
|
|
|
|
|
|
|
*
|
|
|
|
|
1
|
CNP
|
DE-109
|
0,1m
|
|
|
|
|
|
|
|
|
|
|
|
|
|
|
|
|
|
|
|
0
|
CNP
|
|
1,3
|
|
|
|
|
|
|
|
|
|
|
|
|
|
|
|
|
|
|
|
0
|
CNP
|
DE-110
|
0,1m
|
|
|
|
|
|
|
|
|
|
|
|
|
|
|
|
|
|
|
|
0
|
CNP
|
|
1,3
|
|
|
|
|
|
|
|
|
|
|
|
|
|
|
|
|
|
|
|
0
|
CNP
|
D1-101
|
0,1m
|
|
|
*
|
*
|
|
|
|
|
|
|
|
|
|
|
|
|
|
|
|
2
|
CNP
|
|
1,3
|
|
|
*
|
|
|
|
|
|
|
|
|
|
|
|
|
|
|
|
|
1
|
CNP
|
D1-102
|
0,1m
|
|
|
|
|
*
|
|
|
|
|
|
|
|
|
|
|
|
|
|
|
1
|
CNP
|
|
1,3
|
|
|
|
|
|
|
|
|
|
|
|
|
|
|
|
*
|
|
|
|
1
|
CNP
|
D1-103
|
0,1m
|
|
|
|
|
|
|
|
|
*
|
|
|
|
|
|
|
|
|
|
|
1
|
CNP
|
|
1,3
|
|
|
|
|
|
|
|
|
|
|
|
|
*
|
|
|
*
|
|
|
|
2
|
CNP
|
D1-104
|
0,1m
|
|
|
|
*
|
|
*
|
*
|
|
|
*
|
|
*
|
|
|
|
|
|
|
|
5
|
CNP
|
|
1,3
|
|
|
|
|
|
|
|
|
|
|
|
|
|
|
|
|
|
|
|
0
|
CNP
|
D1-105
|
0,1m
|
*
|
|
|
|
|
|
|
|
|
|
|
|
|
|
|
|
|
|
|
1
|
CNP
|
|
1,3
|
|
|
|
|
|
|
|
|
|
|
|
|
|
|
|
|
|
|
|
0
|
CNP
|
D1-106
|
0,1m
|
|
|
|
|
*
|
|
|
|
|
|
|
|
|
|
|
|
|
|
|
1
|
CNP
|
|
1,3
|
|
|
|
|
|
|
|
|
|
|
|
|
|
|
|
|
|
|
|
0
|
CNP
|
D1-107
|
0,1m
|
|
|
|
|
|
|
|
|
*
|
|
|
|
|
|
|
|
|
|
|
1
|
CNP
|
|
1,3
|
|
|
|
|
|
|
|
|
|
|
|
|
|
|
|
|
|
|
|
0
|
CNP
|
D1-108
|
0,1m
|
*
|
*
|
|
|
|
|
|
|
|
|
|
|
|
|
|
|
|
|
|
2
|
CNP
|
|
1,3
|
|
|
|
|
|
|
|
|
|
|
|
|
|
|
|
|
|
|
|
0
|
CNP
|
AN-101
|
0,1m
|
|
|
|
|
|
|
|
|
|
|
|
|
|
|
|
*
|
|
|
|
1
|
CNP
|
|
1,3
|
|
|
|
|
|
|
|
|
|
|
|
|
|
|
|
|
|
|
|
0
|
CNP
|
AN-102
|
0,1m
|
|
|
|
|
|
|
|
|
|
|
|
|
|
|
|
|
|
*
|
|
1
|
CNP
|
|
1,3
|
|
|
|
|
|
|
|
|
|
|
|
|
|
|
|
|
|
|
|
0
|
CNP
|
AN-103
|
0,1m
|
|
|
|
|
|
|
|
|
*
|
|
|
|
|
|
*
|
|
|
|
*
|
3
|
CNP
|
|
1,3
|
|
|
|
|
|
|
|
|
|
|
|
|
|
|
|
|
|
|
|
0
|
CNP
|
AN-104
|
0,1m
|
|
|
|
|
|
|
|
*
|
|
|
*
|
|
|
*
|
|
|
|
*
|
|
4
|
CNP
|
|
1,3
|
|
|
|
|
|
|
|
|
|
|
|
|
|
|
|
|
|
|
|
0
|
CNP
|
AN-105
|
0,1m
|
|
|
|
|
|
|
|
|
|
|
|
|
|
*
|
|
|
|
|
*
|
2
|
CNP
|
|
1,3
|
|
|
|
|
|
|
|
|
|
|
|
*
|
|
|
|
|
|
|
|
1
|
CNP
|
AN-106
|
0,1m
|
|
|
|
|
|
|
|
|
|
|
|
|
|
*
|
|
|
|
|
|
1
|
CNP
|
|
1,3
|
|
|
|
|
|
|
|
|
|
|
|
|
|
|
|
|
|
|
|
0
|
CNP
|
AN-107
|
0,1m
|
|
|
|
|
|
*
|
|
|
|
|
|
|
|
|
|
|
|
|
|
1
|
CNP
|
|
1,3
|
|
|
|
|
|
|
|
|
|
|
|
|
|
|
|
|
|
|
|
0
|
CNP
|
AN-108
|
0,1m
|
|
|
|
|
|
|
|
|
|
|
|
|
|
|
|
|
|
|
|
0
|
CNP
|
|
1,3
|
|
|
|
|
|
|
|
|
|
|
|
|
|
|
|
|
|
|
|
0
|
Total of scarred trees
|
2
|
1
|
1
|
2
|
2
|
2
|
1
|
1
|
3
|
1
|
1
|
4
|
2
|
3
|
6
|
5
|
2
|
2
|
4
|
|
|
+
|
+
|
++
|
|
+
|
+
|
+
|
+
|
+
|
+
|
+
|
++
|
++
|
+
|
++
|
++
|
+
|
+
|
+
|
|
Appendix 5: Fire dating from samples collected
from Upper Aguima Catchment
Trees
|
Height of cutting
|
1995
|
1998
|
1999
|
2001
|
Total
|
A1
|
0,1m
|
|
|
|
|
0
|
A2
|
0,1m
|
|
|
|
|
0
|
A3
|
0,1m
|
|
|
|
|
0
|
A4
|
0,1m
|
|
|
|
|
0
|
A5
|
0,1m
|
|
*
|
|
|
1
|
A6
|
0,7m
|
*
|
|
|
|
1
|
A7
|
0,7m
|
|
|
|
|
0
|
K1
|
0,1m
|
|
|
*
|
|
1
|
K2
|
0,1m
|
|
*
|
|
|
1
|
K3
|
0,1m
|
|
|
*
|
|
1
|
K4
|
0,1m
|
|
|
*
|
|
1
|
K5
|
0,1m
|
|
*
|
|
|
1
|
K6
|
0,1m
|
|
|
|
|
0
|
P1
|
0,1m
|
|
|
|
|
0
|
P2
|
0,1m
|
|
|
|
|
0
|
P3
|
0,1m
|
|
|
|
|
0
|
P7-P8
|
0,1m
|
|
|
|
|
0
|
P9-P10
|
0,1m
|
|
|
|
|
0
|
P11-P12
|
0,1m
|
|
|
|
*
|
1
|
Total of scarred trees
|
1
|
3
|
3
|
1
|
8
|

CURRICULUM VITAE
SINSIN Cocou A. Franck
Beninese, born on September 07, 1983 in
QUALIFICA Comè (Benin).
|
Full address
Phone: (+229) 95 54 14 94
E-mail: sfacsin@yahoo.fr
|
QUALIFICATION
Engineer Agronome, speciality Forestry
PLACEMENT
Postgraduate Student in Lab of Applied Ecology,
FSA-UAC, Benin Researcher in BIOTA-West Africa Programme
KNOWLEDGE
Wood anatomy, Wood physiology, Tree dating methods with special
emphasis on dendrochronology approach, Tropical Ecosystems (Forest and Savanna)
analyses, Tropical ecology, Biodiversity conservation, Tropical silviculture,
Dynamic of Forest, Forest Management, Monitoring approach, Cartography and
SIG
LEARNING AND TRAINING
2008 : Postgraduate student
in Natural Resource management (FSA-UAC, Benin)
2006-2007 : Graduate student
in Natural Resource management (FSA-UAC, Benin)
2002-2006 : Graduate student in
Agronomy (FSA-UAC)
1999-2002 : Second degree of
college, Biology speciality, Benin
1995-1999 : First round of
College, Benin
1989-1995 : Primary
school, Porto-Novo, Benin
DIPLOMAS AND CERTIFICATES
+ 2009
: Master of Science (DEA) in Natural
Resource Management
+ 2008 : Certificate of Deutsch learning
with very good distinction (Göttingen, Germany) +
2007 : Engineer Agronome Degree in
Natural Resource Management (FSA / UAC)
+ 2006 : Degree of General Agronomy
(Faculty of Agricultural Sciences / University of Abomey - Calavi,
Benin)
+ 2002 : A-Levels, option biology
with good distinction (Benin) + 1999 : Brevet of studies
of first college round (Benin)
+ 1995 : Certificate of Primary Education
(Benin)
EXPERIENCES AND PRATICES
+ 1st september to 31 October :
Intern student into Dendrochronology Laboratory
of Institute of Agronomy in the Tropics (Göttingen, Germany).
Application on Using Tree rings analysis to study the growth performance
from sapling to wood of five savanna species (Topic of M -Sc Thesis)
+ 3rd to 31 August 2008 :
Initiation to Deutsch speaking in International Summer
Course (University of Göttingen, Germany)
· :. July to December 2007 : Research in
Lama Forest Reserve on silvicultural and morphological performances of
two sources of Teak ( Tectona grandis L.f). Topic of
Engineer
Agronome Thesis
· . May-June 2006 :
Internship in National wood office of Benin.
· :. March-April 2005 : Monograph
of Rural Area. Identification of constraints for socio
economic development. Applied to village of Assanlin (Zou,
Benin)
+ March 2004 : Methods of Animation and
Intervention in Rural Area (Village of Zoungbomey, Department of
Ouémé): Study the structure and functioning of local
organizations
+ March 2003 : Discovery of Rural Area
(Domanouhoué Village, Town of Aplahoué,
Department of Couffo, Benin): Identification of rural activities
and logic analysis peasant
+ November 2002 : Discovery of
Rural Area. Immersion phase (Village of Dossou somè, Department of
Atlantic, Benin): Making contact with the rural environment
INTERNATIONAL LANGUAGES
+ English: Scientific tongue, Good master in
speaking, in writing and in reading
+ French: Mother tongue
+ Deutsch: Learnt in Germany (International
Summer Course)
INFORMATIC TOOLS
· · Leica and TSAP-win
for Dendrochronology and Wood anatomical
+ Excel, X-act, SAS, Minitab for Data treatment
and Statistical Analysis · · ArcView for
Cartography and SIG
+ Microsoft Word, Power Point for
Presentation
REFEREES
+ Prof. Dr. Ir Brice A. SINSIN : Head of
Laboratory of Applied Ecology and Vice deputy of research in UAC
+ Dr Bettina ORTHMANN : Specialist in wood
Biology, University of Rostock, Germany
SINSIN C. A. Franck
|