FACULTY OF AGRONOMY AND AGRICULTURAL
DEPARTMENT OF AGRICULTURAL ENGINEERING
|
|
FACULTE D`AGRONOMIE ET DES SCIENCES AGRICOLES
DEPARTEMENT DE GENIE RURAL
|
DESIGN OF A GEOGRAPHIC INFROMATION SUPPORTED
DATABASE FOR THE MANAGEMENT OF PRESSURIZED IRRIGATION SYSTEMS AT
THE «PLANTATION DU HAUT PENJA» (PHP),
NJOMBE (CAMEROON)
By
CHICK Herman AZAH
Registration N°: 04A012 Major: Agricultural
Engineering
Thesis presented in partial fulfillment of the requirement
for the award of the «Ingénieur Agronome» Diploma
CO-SUPERVISOR: Mr. Njila Roger,
MSc Assistant Lecturer, Dep`t of Agric. Engineering
FACULTY OF AGRONOMY AND AGRICULTURAL SCIENCES
DEPARTMENT OF AGRICULTURAL ENGINEERING
|
|
FACULTE D`AGRONOMIE ET DES SCIENCES AGRICOLES
DEPARTEMENT DE GENIE RURAL
|
DESIGN OF A GEOGRAPHIC INFORMATION SUPPORTED
DATABASE FOR THE MANAGEMENT OF PRESSURIZED IRRIGATION SYSTEMS AT
THE «PLANTATION DU HAUT PENJA» (PHP),
NJOMBE (CAMEROON)
By
CHICK Herman AZAH
Thesis presented in partial fulfillment of the requirement
for the award oi lIKE ,« gpnilAr SRRnRPH El iSCRPa
ACADEMIC SUPERVISOR: Prof. Mathias Fru Fonteh,
PhD
Associate Professor, Dep`t of
Agric. Engineering
FIELD SUPERVISOR: Mr. Boa Appolinaire,
Eng Irrigation Department, PHP Njombé
CERTIFICATION OF ORIGINALITY OF THE WORK
This is to testify that, the work in this thesis was carried
out by CHICK Herman AZAH at the Plantation du Haut
Penja (PHP) under the field supervision of Mr. Boa
Appolinnaire and academic supervision of Prof. FONTEH Mathias
Fru and Mr. Njila Roger. The work is original and has not been
presented for the acquisition of a university degree or diploma elsewhere.
Names and Signatures of supervisors Name and Signature
of author
Prof. FONTEH Mathias Fru CHICK Herman AZAH
Date: Date:
Mr. NJILA Roger
Date:
CERTIFICATION OF CORRECTION OF THESIS
This thesis has been revised and corrected in conformity to the
modifications suggested by the examination panel of
Signature of Supervisor Signature of
Candidate
Date Date
Signature of Member of Jury Signature of Member of
Jury
Date Date
Signature of Member of Jury Signature of President of
Jury
Date Date
Signature of Head of Department
Date
ABSTRACT
Most of the data related to irrigation systems can now be
characterized geographically. A study geared towards the creation of a
geographic information supported database for the management of pressurized
irrigation systems was carried out at the Plantation du Haut Penja?. The
irrigation system of this group is becoming very complex and diversified due to
the increase in the number of hectares of banana cultivated each year. The
putting in place of new systems, management and monitoring of this system is
thus subjected to several constraints. The specific objectives of the study
were to: develop a database for rapid access and orderly storage of information
regarding the irrigation system; develop thematic layers for the GIS, evaluate
the water requirements in each plot; evaluate the functioning of the networks
and to spatially represent some aspects of the irrigation system. The database
on the irrigation system was created using Microsoft Access 2003 while the
various layers of the GIS were created using MapInfo 8.0 software. An Object
Database Connection (ODBC) was created between the MS Access database and the
MapInfo GIS to carry out multiple queries on the irrigation system and
spatially represent some aspects of the irrigation system. A total of 35
tables, 10 forms and 17 queries were created for the database to enhance data
entry and retrieval. The probability of satisfaction of crop water requirements
for a 20 years climatic data was calculated. The crop water requirement for an
effective root depth of 50 cm was 40 mm/week for the satisfaction of the crop
water requirements 1 out of 20 years. Depending on the crop evapotranspiration
and effective rainfall of the previous day, the crop water requirements will be
adjusted in the database. Thematic layers for the GIS such as the crop
varieties, spatial arrangement of the crops, soil types, type of irrigation
system, plot of valves and others were created in the database. Some of these
thematic layers were represented spatially using MapInfo 8.0 to demonstrate the
use of the GIS when coupled to the database. Analysis of the flow rates,
pressures, flow velocities and other hydraulic properties of the network showed
these to be within the limits of hydraulic flow in pipes. This indicates that
more emphasis should be laid on monitoring and management of this system;
hence, the necessity of the GIS database developed in this study in order to
ameliorate the management of this system.
RESUME
La plupart des données relatives aux systèmes
d'irrigation peuvent maintenant être caractérisés
géographiquement. Une étude orientée vers la
création d'une base de données géographiques (SIG) pour la
gestion des systèmes d'irrigation sous pression a été
effectuée à la "Plantation du Haut Penja". Le système
d'irrigation de ce groupe devient très complexe et diversifié en
raison de l'augmentation du nombre d'hectares de bananiers cultivés
chaque année. Les objectifs spécifiques de l`étude
étaient de: développer une base de données pour un
accès rapide et un stockage méthodique des informations relative
a ce système d'irrigation; développer des couches
thématiques pour le SIG; évaluer les besoins en eau de chaque
parcelle; évaluer le fonctionnement des réseaux et de
représenter spatialement certains aspects du fonctionnement du
système d'irrigation. La base de données sur le système
d'irrigation a été créée à l'aide de
Microsoft Access 2003 tandis que les différentes couches du SIG ont
été créées à l'aide du logiciel MapInfo 8.0.
Une connexion objet de base de données (ODBC) a été
créé entre la base de données MS Access et le logiciel SIG
MapInfo pour effectuer des requêtes sur le système d'irrigation et
représenter certains éléments spatiaux du système
d'irrigation. Un total de 35 tables, 10 formulaires et 17 requêtes ont
été créés pour la base de données afin
d'améliorer la saisie et la récupération des
données. Le calcule de la probabilité de satisfaction des besoins
en eau des cultures de 20 ans de données climatiques pour une profondeur
effective des racines de 50 cm, ces besoins étaient de 40 mm/semaine
pour une satisfaction des besoins en eau des cultures 1 an sur 20. En fonction
de l'évapotranspiration des cultures et de l'efficacité des
pluies de la veille, les demandes en eau des cultures seront ajustées
dans la base de données. Les couches thématiques pour les SIG,
telles : les variétés de cultures, la disposition spatiale des
cultures, types de sols, le type de système d'irrigation, la
répartition des précipitations et d'autres ont été
créés dans la base de données. Certaines de ces couches
ont été représentées spatialement en utilisant le
logiciel MapInfo 8.0. L'analyse des débits, pressions, vitesses
d'écoulement et d'autres propriétés hydrauliques du
réseau a montré que les conditions limites d`écoulement
dans les conduites sont respectées. Cela indique davantage qu`un accent
devrait être mis sur la gestion de ce système ; d`où la
nécessité d`un outil tel le SIG développé dans le
cadre de la présente étude en vue d`améliorer la gestion
du système.
ACKNOWLEDGEMENTS
Knowledge is like a cult which quickly withers away when there
are no disciples, comforters nor supporters. It is in this like that I will
like to appreciate those who have been instrumental to me during this period of
scholastic rummaging. This list is a non exhaustible one which I`ll like to use
to show appreciation:
To the Almighty God Who has given me the opportunity to become
what I am today and who has guided and directed me all the days of my life.
To my supervisors, Prof. Fonteh Mathias who has always guided
me in most of my academic work and Mr. Njila Roger who put efforts together to
see that this work becomes a reality and for the time they visited me on the
field. I`ll forever be grateful to them for the knowledge on database
management they`ve imparted on me.
To my field supervisor, Mr. Boa Apollinaire, thank you
wouldn`t just be enough for me to offer you. You gave in all you could for me
to carry out my internship without stress and you were always in there to guide
me, sometimes till late at night. This and many other things you did for me
during this six month period are enough reasons for me to be thankful.
To Mr. Tsimi Hiliare Zoa, Director of Human Resources at PHP
for the partnership created with the department of agricultural engineering
which gave rise to this internship. This goes a long way to show the
contribution of your company to the training of young Cameroonians in the
agricultural sector.
To Mr. Jean Yves Regnier, Mr. Tchoumba Jules, Mr. Ndosse
Robert, Mrs. Guenaelle Renovolt, Mr. Andjengo Emmanuel all senior workers of
PHP, for the technical advice I received from them during this period and for
all the logistics they provided me with.
To Dr Berinyuy Joseph and Mr. Tekounegning whose comments have
always been very valuable, I owe much honour.
To the lecturers of the Faculty of Agronomy and Agricultural
Sciences and especially those of the Department of Agricultural Engineering,
who have inculcated into me much knowledge in the domain of agronomy.
To Mr. Marin Mahop, of the Department of Agricultural Engineering
for his availability in giving us the technical advice we`ve always needed
during this period.
To Prof. Ajaga and family, Dr. Focho and Family, Fonteh`s
Family, Dr. Ayissi and family for their wise counsel, advice and for moral
encouragements.
To my mates and friends of the 12th batch of FASA
and particularly those of the department of agricultural engineering, for the
wise complements and advice bestowed on each other during these years we`ve
spent together.
To my very dear friends Muyang Achah, Etubo Constance, Ngalim
Olive, Chiato Maryben, Eseinei Paul, Tenkang Ernest, Kilain Fru, Lebaga Eloy,
Tarla Divine, Fointama Nyongo, with whom I had much joy and struggles during my
course period in Dschang.
To Mr. Ewome Hardison for the hospitality he showed me all
through my stay with him in Njombé. Mr. Fongoh Wilson and Family, Mr.
Fonbah Cletus, Mr. Fondi Emmanuel for making me not feel homesick during this
period of intense stress.
DEDICATION
To my parents Mr. and Mrs. Azah who have relented none of their
efforts in seeing me through my educational career and to whom I owe much
To my brothers, Teku Elvis and Boubga Clifford and my sister,
Wansho Gilda for the
love and solidarity that we express towards each other
To Late Dr. Ambe Fokwa, for all the advice I received from him
during my years of work with him at the Department of agricultural Engineering
and may He find Profound Peace in the Almighty
TABLE OF CONTENTS
LIST OF TABLES xii
LIST OF FIGURES xiii
LIST OF ABBREVIATIONS, ACRONYMS AND SYMBOLS xiv
CHAPTER I INTRODUCTION 1
1.1 Background of the Study 1
1.2 Problem Statement 3
1.3 Objectives of the Study 4
1.4 Importance of the Study. 5
CHAPTER II LITERATURE REVIEW 7
2.1 Banana 7
2.1.1 Introduction 7
2.1.2 Ecology 8
2.2 Definition of some Terms and Concepts related to Irrigation
10
2.3 Evapotranspiration 13
2.3.1 Measurement of evapotranspiration 15
2.3.2 The Penman-Montheith equation 15
2.3.3 Meteorological factors determining evapotranspiration 16
2.4 Maximum Production 18
2.5 Pressurized Irrigation Systems 19
2.5.1 Sprinkler irrigation systems 19
2.5.2 Micro irrigation systems 20
2.6 Irrigation Scheduling and Management 21
2.6.1 Irrigation management 21
2.6.2 Irrigation scheduling 21
2.6.3 Importance of irrigation scheduling 24
2.7 Geographic Information Systems 24
2.7.1 Data acquisition and representation 26
2.7.2 Advantages and disadvantages of vector and raster data
28
2.7.3 Steps used for the putting in place of a GIS project 29
2.8 Databases 30
2.8.1 Database management systems 32
2.8.2 Relational databases 33
CHAPTER III MATERIALS AND METHODS 36
3.1 Description of the Study Area and Experimental Site 36
3.1.1 Geographical Location 36
3.1.2 Relief 36
3.1.3 Hydrology 36
3.1.5 Vegetation 39
3.1.6 Climate 39
3.1.7 Soils 40
3.2 Description of the Irrigation System at the PHP Group 40
3.2.1 Pumping station 41
3.2.2 The main line (Pipes) 41
3.2.3 Distribution network 43
3.3 Development of the Database for the Irrigation System 44
3.3.1 Data review 45
3.3.2 Entity and attribute identification 45
3.3.3 Table and key creation 46
3.3.4 Definition of relationships and referential integrity 47
3.3.5 Creation of data entry and retrieval forms 48
3.4 Development of thematic layers for the GIS 50
3.5 Calculation of the water requirements in each plot 51
3.5.1 System requirements 51
3.5.2 Crop requirement 52
3.6 Evaluation of the Functioning of the Network 53
3.6.1 Calculation of flow rates 54
3.6.2 Calculation of flow velocity and head losses. 54
3.6.3 Determination of available and required pressures 55
3.6.4 Calculation of piezometric elevations 57
3.7 Spatial Representation of some Aspects on the Irrigation
System 58
CHAPTER IV RESULTS AND DISCUSSIONS 60
4.1 Database for the Irrigation System 60
4.1.1 Physical model 60
4.1.2 Creation of forms 62
4.2 Thematic layers for the GIS 63
4.3 Water requirements in each plot 66
4.3.1 System requirements 66
4.3.2 Crop water requirements 67
4.4 Simulation of the Functioning of the Network 69
4.5 Spatial Representation of some Queries on the Irrigation
System 70
4.5.1 Spatial representation of crop coefficients 70
4.5.2 Spatial representation of some plot valves 71
4.5.3 Theissen polygon for rainfall heights on the plantation
72
CHAPTER V: CONCLUSIONS AND RECOMMENDATIONS 74
5.1 Conclusions 74
5.2 Recommendations 75
5.2.1 Improvement of the system 75
5.2.2 Further research 75
REFERENCES 76
APPENDICES 82
LIST OF TABLES
Table 2.1
|
Length of crop growth developmental stages for various planting
periods
|
Pages
|
|
and Climatic regions
|
14
|
2.2
|
Monthly KC values of Banana for tropical climate
|
15
|
2.3
|
Set of related fields in an irrigation system which form a record
|
31
|
2.4
|
Comparing DBMS and Relational DBMS (RDBMS) terms
|
32
|
3.1
|
Average annual precipitation of Njombé (2004-2008)
|
39
|
4.1
|
Thematic layers needed for water balance calculations
|
63
|
4.2
|
Thematic layers for non-descriptive data
|
64
|
4.3
|
Thematic layers for non-descriptive data ...
|
65
|
4.4
|
Probability of satisfaction of irrigation requirements
(requirements in mm)
|
68
|
4.5
|
Irrigation dose (mm) for two irrigation systems.
|
69
|
LIST OF FIGURES
Figure
|
Pages
|
2.1
|
Morphology of a banana plant...
|
8
|
2.2
|
One-to-one relationship of databases.
|
35
|
2.3
|
One-to-many relationship of databases.
|
35
|
3.1
|
Geographical location of Njombé
|
37
|
3.2
|
Aerial view of PHP cultivation areas in the Njombé
Plantations
|
38
|
3.3
|
Monthly rainfall histogram for Njombé in 2008
|
40
|
3.4
|
Principal Irrigation Pipes at the PHP group
|
42
|
3.5
|
Architecture of the GIS database
|
45
|
3.6
|
Creation of table in design mode in MS access
|
46
|
3.7
|
Definition of relationships in the physical data model
|
.48
|
3.8
|
Selecting fields to be included in the production plot form under
the form
|
|
|
assistant mode
|
..49
|
3.9
|
Irrigation map for a production plot developed with AUTOCAD 2004
|
51
|
3.10
|
Query created in MS access to obtain the water requirements of
the system
|
52
|
4.1
|
Presentation of the physical model of data as developed in MS
Access
|
.61
|
4.2
|
Form for data entry and retrieval for the production plot
|
.62
|
4.3
|
System water requirement as calculated in MS access
|
.66
|
4.4
|
Sensibility of various plots to water stress with respect to
Kc
|
71
|
4.5
|
Plot valves for two irrigation plots ...
|
...72
|
4.6
|
Repartition of rainfall depths in the plantation
|
73
|
LIST OF ABBREVIATIONS, ACRONYMS AND SYMBOLS
BLOB: Binary Large Object
cp: Specific heat of the air
CSQL: Compact Standard Query Language
D: Zero plane displacement height [m],
DBMS: Database Management System
ea : Actual Vapour Pressure [KPa]
es: Saturation Vapour Pressure[KPa]
ESRI: Economic and Social Research Institute
G: Soil heat flux
GIS: Geographic Information System
INGRES: Intelligent Graphic Relational System
JPEG: Joint Photographic Experts Group
P: Depletion factor
PHP: Plantations du Haut Penja
PS: Photosynthesis
ra: Aerodynamic Resistance [sm-1],
RAW: Readily Available Water
RDBMS: Relational Database Management System
RGB: Red, Green, Blue
Rn: Net solar radiation
rs: Bulk? Surface Resistance
SPM : Société des Plantations de Mbanga
SQL: Standard Query Language
TIF: Tagged Image File
ã: Psychometric Constant
Ä: Slope of the saturation vapour pressure temperature
relationship
ña: Mean air density at constant pressure
Zm height of wind measurements [m],
Zh height of humidity measurements [m],
Zom: roughness length governing momentum transfer [m],
Zoh: roughness length governing transfer of heat and vapour
[m],
K: Von Kerman`s constant, 0.41 [-],
Uz: wind speed at length at length z
[ms-1].
CHAPTER I INTRODUCTION
1.1 Background of the Study
Bananas are presently the world`s fourth most important food
commodity in terms of gross value of production (Lemeilleur et al.,
2003). Banana cultivation is a major source of foreign exchange and
continues to be one of the principal agricultural activities for most
developing countries of Africa, Latin America and the Caribbean. World
production of bananas (dessert and plantain bananas) is estimated at some 40 to
60 million tons. Some 7-8 million tons (mostly dessert bananas) are exported to
the developed countries yearly (Pedro et al., 2003). The banana
industry has been designed and oriented almost exclusively towards the export
market (Yamileth, 1998). As merchandise for exportation, bananas contribute
principally to the economy of a number of countries with low income, such as
Ecuador, Honduras, Guatemala, Cameroon, Ivory Coast, and the Philippines (Pedro
et al., 2003).
About 700 000 tons of bananas are produced annually in
Cameroon by three main companies: the Plantation du Haut Penja? (PHP) Group,
Del Monte, and the Société des Plantations de Mbanga? (SPM)
(Anonymous, 1998). This production yielded 103 billion FCFA during the
2001-2002 financial years for an investment of 12 billion 108 million FCFA
(Anonymous, 2003).
The production of this crop at an industrial scale entails the
use of much water. Farms require water for irrigation in the dry season and
packing stations use water for washing bananas. Fonteh and Assoumou (1996)
describe irrigation as the supply of water to crops in a climate in which
rainfall does not meet the crop water requirements during all or part of the
growing season. Tiercelin (1997) defines irrigation as the artificial use of
water to ameliorate yields or crop production. The same author states that more
than one-third of the world`s food is produced through irrigated agriculture.
About 280 million ha of land are irrigated around the world with an annual
increase of four to five thousand hectares yearly (Rieul et al.,
1992).
Irrigation could be total or supplemental. In total
irrigation, provision is made for all plant water needs. This is the case in
regions where no rainfall can be relied upon during all
or part of the crop growing season. Supplemental irrigation is
practiced in areas where a crop can be grown by natural rainfall alone, but
additional water improves yields and quality (Fonteh and Assoumou, 1996). The
following are reasons why crops could be irrigated:
1. Supply water for plant growth where none could grow before or
to get better growth or extend the growing season, all leading to increased
yields.
2. To improve quality (Robinson, 1981).
3. As an insurance policy against drought such that if water
will affect the returns on high investments on seeds, fertilizers, etc., then
irrigation is planned for.
4. Sprinkler irrigation is used for temperature control:
· Frost protection: in very low temperatures, the water
from a sprinkler on plants freezes, giving off the latent heat of fusion.
· Evaporative cooling: in hot weathers, water from
sprinklers evaporates, absorbing the latent heat of evaporation form the
atmosphere around the plants, leading to a drop in temperature.
5. To leach unwanted salts building up in the top soil.
6. Reduce soil strength at the start of the dry season for
easy cultivation.
7. For the application of chemicals (chemigation) or fertilizers
(fertigation). Robinson (1981) presents the following specific advantages of
irrigating bananas:
· Well irrigated banana plants have turgid pseudostems, are
vigorous, with a high resistance to wind and diseases;
· Irrigation favors the application of fertilizers
especially during dry periods;
· The life span of an irrigated banana plot is higher than
that of a non irrigated plot;
· Irrigation promotes the continuous production cycle of
bananas;
· Irrigation improves the quality of fruits, increases
the length and width of banana fingers, helps in obtaining higher grades and in
the development of large bunches (15 to 18 hands).
In Cameroon, bananas are irrigated during the dry seasons.
During these periods, there is little or no rainfall to provide the plant water
requirements (Ewane, 2008). Irrigation is therefore resorted to as a means of
supplying the crop water requirements and
to improve the yield and quality of bananas during these dry
periods. Robinson (1981) showed that yields increase by 20-30 tons per hectare
of banana in Natal, South Africa when additional water was supplied at fourteen
days intervals. Further increase from 60-80 % in extra quality was recorded
compared to non- irrigated banana plantations. Three forms of irrigation are
currently practiced in banana plantations around the world namely; surface
irrigation, sprinkler and drip irrigation (Stover and Simmonds, 1987). Trials
in South Africa have shown that drip and micro sprinkler irrigation systems
have each outperformed the others irrigation systems in yields and in water
economy (Robinson and Alberts, 1987).
Many new technologies, such as remote sensing, geographic
information system (GIS) and expert system, are now available for application
to irrigation systems and can significantly enhance the ability of water
managers (Mennati et al., 1995, Ray and Dadhwal, 2001).
There exists a global water crisis in the world in this
century. Conscious of the situation of water crisis and other alarming
statistics around the world, the Plantation du Haut Penja (PHP) attaches
importance to the efficient management of its water resources. AQUASTAT (2009),
for example, shows that, of the total water available on the earth`s surface,
97.5% is salt water and only 2.5% is fresh water. Of this 2.5% freshwater, 99%
is locked up in glaciers, icebergs or underground and only 1% is available to
the nearly seven billion humans and billions of other forms of life. It further
gives a closer look to the situation in the Lake Chad area, which was once a
landmark for astronauts circling the earth, but now difficult to locate.
Surrounded by Cameroon, Chad, Niger, and Nigeria, the lake has shrunk by 95 %
since the 1960s (AQUASTAT, 2009). The soaring demand for irrigation water in
this area is draining dry the rivers and streams the lake depends on for its
existence. As a result, Lake Chad may soon disappear entirely, its whereabouts
a mystery for future generations. With this limited freshwater resource and the
increasing competition for the resource, irrigated agriculture worldwide must
improve the utilization of these water resources (Molden et al.,
1998).
1.2 Problem Statement
The sprinkler irrigation system at the PHP Group, Njombé,
covers a surface area of about 3500 ha (Boa, 2005). The putting into place,
management and monitoring of this
system is subjected to several constraints which are becoming
more and more difficult to handle if one is to consider:
· the diversity and complexity of the irrigation
networks;
· the simultaneous exigencies of water on various plots
with different sensibility to water stress, and the incapacity of the system to
meet these needs at once by operating as scheduled;
· pressure from international organizations, such as the
European Union, to show improved water use efficiency, increasing the necessity
to record, organize and present large amounts of data that were not originally
needed for day-to-day operations,
· the exigencies on water economy, with the strict
application of the law on the use of water resources in Cameroon.
Several equipment and materials are used in the irrigation
networks of this company. The qualities as well as the performance level of
irrigation materials are essential factors in the efficiency and durability of
the systems which they constitute (Adam & Beaudequin, 1997). However, the
management, the conditions of use of these equipments, and the percentage of
the energy component used in the transport and distribution of water
constitutes 70-75% of the irrigation cost (Thomé, 2007). The system no
longer provides the crop water requirements during peak periods because of the
low flow rates required by all the hydrants expected to operate simultaneously.
This therefore leads to a situation of non satisfaction. Because of this non
satisfaction, only few hydrants situated downstream can be operated at the
detriment of the others for which the flow rates and pressures become
insufficient. The irrigation managers therefore, usually adopt only some
empirical methods for the distribution of water, with their most important
rationale being the reduction of energy consumption without necessarily
providing the crop water requirements. The areas eventually irrigated are often
less than planned, efficiencies are lower and crop yields are not as high as
expected.
1.3 Objectives of the Study
The goal of this study is to develop a GIS supported database
which will be a tool for the improvement of water management techniques and
irrigation scheduling; providing
better decisions for irrigation managers. Elimination of
deficiencies of management and organization is seen as an important tool in
solving problems of water management in irrigation systems. Monitoring and
evaluation are therefore, getting more importance in irrigation management
(Sisodia, 1992). Many studies indicate that, the main reason for poor
performance of irrigation systems is the lack of efficient irrigation
management rather than technical deficiencies (Sisodia, 1992). Information
which should help system managers should thus be easily accessible in
irrigation management.
The specific objectives of the study will be to:
· Develop a database for rapid access and orderly storage
of information regarding the irrigation system,
· Develop thematic layers for the GIS,
· Calculate the water requirements in each plot,
· Evaluate the functioning of the irrigation network,
· Spatially represent the main aspects with regards to the
irrigation system.
1.4 Importance of the Study.
The GIS will provide a means of measuring spatial and
attribute data in a computerized database system, thereby allowing input,
storage, retrieval and analysis of geographically referenced data. It will
analyze spatial interactions between static and dynamic entities and will be a
simulation tool for actual field situations.
Spatial representation of the results will help in the
localization of disfavored branches during irrigation periods. This will lead
to the adoption of management rules and the scheduling of interventions at the
level of the system (reinforcement, reduction of flow rates...) if necessary.
However, spatial representation of periodic data for performance parameters
compared to crop water requirements will help irrigation managers to locate
over consumption, water loss in the plot and other eventual problems in the
system.
The study will help in the development of better water
management options of maximizing profits on capital invested. This is because
the cost of irrigation ranks first on the cost items of the company.
To some extent, the GIS would help in the management of other
farm operations such as fertilization, planting, and harvesting as the database
created contains tables with
information on the agronomic state of production plots. The
management of these operations could thus be represented spatially for better
monitoring.
On the socio economic point of view, amelioration of water
management techniques and an increase in profits will probably lead to more
area being put under cultivation thereby increasing the number of jobs in the
Njombé-Penja area.
The study is a step towards the generation of information
necessary for managing water efficiently.
CHAPTER II LITERATURE REVIEW
2.1 Banana
2.1.1 Introduction
Banana is a monocotyledon of the Order: Scitaminales, Family:
Musa, Sub-family: Musoideae (Stover and Simmonds, 1987). Valmayor (1991) used
15 plant morphological characters to score commercial banana cultivars into one
species or another. Stover and Simmonds (1987) distinguish two banana species,
namely Musa acuminata and Musa balbisiana. Commercial
cultivars are mainly triploids of the genus Musa (Lane, 1955). The cultivated
cultivar of the sub-tropics is of the Cavendish sub-group. This sub-group is
made up of the Grand Nain (GN) and the Williams cultivars with the Williams
cultivar gaining more popularity due to its hardiness, superior bunch
conformation and ease of packing.
Banana is a herbaceous plant; it has an upper pseudostem and
an underground part. The upper trunk could have heights ranging from 1.5-8 m
depending on the species (Lassoudiere, 1979; Stover, 1979). The average heights
of cultivated cultivars could be as short as 1.5 m in dwarf plants or as tall
as 8 m in a ratoon crop of GN or W cultivars. The root system is fleshy and
adventitious. Horizontal and vertical distribution of roots is strongly
influenced by soil type, compaction and drainage (Riopel & Steeves, 1964;
Summerville, 1939). Horizontal extension of primary roots is usually between
1-2 m but can be as long as 5 m (Robinson, 1987). The vertical root zone is
very shallow with 40 % of the root volume in the top 200 mm and 85 % in the top
300 mm of soil. However, effective root depth for irrigation purposes stands at
500 mm. Mature leaf length is between 1.8 m and 2.4 m, with a width of about 1
m. A vigorous ratoon plant has about 24 m2 functional leaf area at
its morphological peak. Fruits are formed on =hands` with about 12 fingers;
each bunch can carry up to 150 fingers. After harvest, the pseudo stem is cut
down.
Three banana production systems can be distinguished: the
traditional, semi traditional and modern systems of production. All these three
systems are practised in Cameroon, supplying the local and world markets with
banana (Fonsah and Chidebelu, 1985). PHP group practices the modern system
which entails much care. The agricultural
activities carried out include: nursery and soil preparation,
planting, propping, pruning, weed and pest management, fertilizer application,
deleafing, fruit care, selection of suckers, replanting, harvest and
exportation (Robinson and De Villier, 2007).
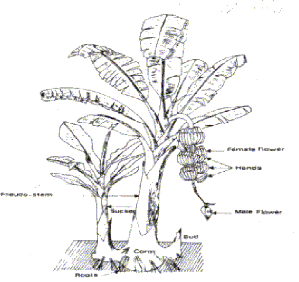
Source: Robinson and De Villier, 2007 Figure 2.1:
Morphology of a banana plant
2.1.2 Ecology
Banana is cultivated principally in the tropics. The major
banana-growing regions of the world are situated between the equator and
latitudes 20°N and 20°S. The crop has a high water demand and is
sensitive to low temperatures and wind. The principal environmental factors
which affect the growth of banana are:
· Water
Availability of water is one of the critical factors that
determine where bananas should be grown. It is generally considered that
bananas require a weekly precipitation of 30-40 mm of rainfall or 1500-2000 mm
annually for optimum growth. There is however, overwhelming evidence worldwide
(except in parts of the humid tropics) to support the need for supplementing
irrigation of bananas as rainfall distribution is seasonal and erratic.
With respect to the use of water, the banana plant has a number
of important characteristics (Swennen and Vuysteke, 2001):
- A high evapotranspiration rate due to large broad leaves and
large total surface area. Maximum evapotranspiration is estimated at between
5-6 mm/day.
- A shallow superficial root system compared with most
tree-fruit crops. In general, 100 % of water is obtained from the first
0.5-0.8 m with 60 % from the first 0.3 m.
- A poor ability to withdraw water from a soil with low
moisture content. A depletion of 35 % (management allowable deficit) of the
total available water should thus not be exceeded.
- A rapid physiological response to soil water deficit
especially in conditions of high evaporative stress. Robinson & Alberts
(1987), found that, after 6 days without water, when tensiometers showed 25
kPa, the level of photosynthesis on banana plants reduced by 19 % compared with
well-watered plants. When tensiometers showed 70 kPa, the level of PS was
reduced by 80 %. At this stage, external wilting symptoms are clearly
visible.
· Temperature
The rate of banana growth and development is determined by
temperature. On the basis of the mean daily temperatures (maximum + minimum
/2), the optimum mean for photosynthesis and flower initiation is 22°C,
whereas the optimum mean for plant development and leaf emergence is 31°C
(Turner & Lahav, 1983; Robinson & Anderson, 1991). Mean temperature
balance required for growth (assimilation) and development (leaf emergence) is
27°C.
· Soils
According to Delvaux (1995), soil physical factors important
for banana are porosity and mechanical impedance (compaction), aeration and
natural drainage (water logging), water-holding capacity and soil temperature.
Plantation longevity and sustained high production is dependent on porous,
loose soils which allow unimpeded root extension. Banana root density is thus
inversely related to soil bulk density. When using a penetrometer, the soil
strength should not exceed 1500 kPa down to 800 mm depth. Studies in
waterlogged soils, in South Africa indicate drains should be dug between rows
of bananas to assist in the removal of excess water from about 12 m on both
sides of the drain,
thus a deep drain every eight banana rows will be a useful
insurance policy (Robinson and De Villier, 2007). Minimum soil temperatures of
10°C to 15°C are severely restricting on banana root extension.
Hence, the slope aspect which conditions the field exposure to sunlight as well
as the planting density will affect soil temperature.
Sandy Clay soils are best for bananas because there is a good
balance between the water-holding capacity and the cation exchange capacity on
the one hand, and increased aeration, water infiltration and drainage on the
other hand. Optimum soil texture should be about 30% clay, 10% silt and 60%
sand. The texture thus determines the total available water (TAW). The TAW is
expressed in mm water/m soil depth. Light sandy soils will therefore require
more frequent watering to maintain field water capacity than will do loam or
clay soils.
Soil chemical aspects such as soil acidity and salinity are
equally important for plant growth and in irrigation management (Robinson and
De Villier, 2007). For optimum plant growth, the soil pH measured in water
should be between 5.8 and 6.5. Salinity is usually only a problem in
Mediterranean climates, which have saline soils, low rainfall and use poor
irrigation water. Thus, soils with electrical conductivities of less than
1mmho/cm are required for good growth.
· Wind
Wind causes different types of damage in banana plantation.
At wind velocities of more than 70 km/h, between 50-100% of the plants can be
blown down. Winds modify the physiological functioning of the banana plant
through its effect on the boundary layer of moist, undisturbed air adjacent to
the leaf surface, and by its effect on leaf temperature. If the wind speed is
high and humidity low, the boundary layer quickly disperses, leaf temperature
rise, stomata close and the plant suffers physiological stress. Propping is
usually employed in supporting banana and windbreak and hedges to prevent wind
damage.
2.2 Definition of some Terms and Concepts related to
Irrigation
Field Capacity (FC)
This is refers to the maximum quantity of water that the soil
can hold against the forces of gravity. It corresponds to a suction of 0.1bar
(Fonteh & Assoumou, 1996).
Permanent Wilting Point (PWP)
This is the moisture content at which a plant wilts
permanently under conditions of water stress even if it is later placed in a
saturated atmosphere. It is assumed to correspond to a suction of 15bars
(Fonteh & Assoumou, 1996).
Available Water Content (AWC) and Total Available Water
Content (TAWC)
This is the quantity of water that is readily available in a
soil for plant growth. It is expressed as the difference between field capacity
and permanent wilting point.
AWC= èfc - èwp (2.1)
The total Available Soil Water content (TAW) is defined as
the difference in soil moisture content between soil field capacity
(èfc) and wilting point
(èwp). It represents the ultimate amount of
water available to the crop and depends on the texture, structure and organic
matter content of the soil. The total available water in the root zone can be
calculated as follows (Hanks and Ashcroft, 1980):
TAWC = (èfc - èwp) Zr
(2.2)
Where,
TAWC= Total available soil water in the root zone (m)
èfc = Water content at Field capacity
(m3/m3)
èwp = Water content at wilting point
(m3/m3)
Zr = Root Depth (m)
Root depth growth with time can be calculated using the
procedure described by Borg and Grimes (1986) and given by the equation:
Zr = Zrm [0.511 +0.511Sin (3.03 - 1.47)]
(2.3)
Where,
The angle is in radians,
Zr is the root depth in cm,
Zrm is the maximum root depth of the crop in cm, DAP is the
number of days after planting, and
DTM is the number of days to maximum root depth.
The root depth growth rate is 1.2 mm/day for grass and 1.5
mm/day for banana until maximum effective root depth has been reached (Plauborg
et al., 1996). The maximum effective root depth is determined by both
crop and soil type.
Soil Moisture Deficit (SMD)
This is the difference between field capacity and the actual
soil moisture content. It is normally the depth of water that should be
replaced by irrigation (Merriam & Keller,
1975).
P
eff o
Effective Rainfall
? 125
This is the fraction of precipitation that is effectively
used by plants after the deduction of surface run off and deep percolation (Van
Laere, 2003). The effective precipitation depends on a number of variables:
amount, intensity and frequency of rainfall; evaporative demand; terrain
characteristics; soil and crop; groundwater location; management practices;
etc., (Kopec et al., 1984). Due to the difficulty of measuring all
these variables, some authors recommend the use of empirical equations or to
estimate the effective precipitation (Peff) as a percentage of total
precipitation (Ptot). In the last case, a value of 80 % is
recommended when rainfall depth is below 100 mm/month (Rojas & Rolda'n,
1996). Moon and Van der Gulik (1996) stated that the effective precipitation is
ignored if it is under 5 mm/day as this amount is not likely to penetrate the
soil surface and will be evaporated. The effective rainfall could equally be
calculated as proposed by the United States Department of Agriculture Soil
Conservation Service (Smith et al, 1996).
for Ptot < 250mm (2.4)
for Ptot > 250mm (2.5)
Readily Available Water and Depletion Factor
The fraction of total available water that a crop can extract
from the root zone without suffering water stress is the readily available
water (RAW). The depletion factor is the fraction of the total available soil
water that can be depleted from the root zone before moisture stress occurs (P
ranges from 0 to 1). The P values are expressed as a fraction of TAWC with
lower values taken for sensitive crops with limited root systems under high
evaporative conditions, and higher values for deep and densely rooting crops
and low
evaporation rate (Doorenbos et al., 1986). At low
rates of ETc, the p values are higher than at higher rates of Etc.
RAW = P * TAWC (2.6)
Where,
RAW = Readily Available Water,
P= depletion factor (0.35 for banana plants).
2.3 Evapotranspiration
One way to improve water use efficiency and optimize plant
production is to provide crops only with the water they need based on the
climate-plant-soil relationship. Therefore the concept of evapotranspiration
(ET) is the base for the right amount of irrigation water that should be
applied.
Water supplied to crops is lost from the soil through direct
evaporation and transpiration into the atmosphere (Fonteh and Assoumou, 1996).
It is difficult for us to isolate the two mechanisms on a field with growing
crops. As such the two losses are usually combined to give evapotranspiration
(ET). Knowledge of ET enables us to predict the soil moisture deficit (SMD) for
irrigation. We express the ET as a rate of loss of water e.g. 5 mm/day. ET
could also be referred to as the consumptive use i.e. the total amount of water
a crop takes from the soil as it grows. Designs of irrigation systems are
usually based on the period of the growing season with the maximum consumptive
use. Systems are designed with capacities to supply water when demand is the
highest. We can differentiate between two types of ET: reference crop ET and
actual ET. Reference crop evapotranspiration (ETo) is the water use
of a vigorously growing reference crop under full cover, when water is not
limiting (Fonteh and Assoumou, 1996). The crop or actual evapotranspiration
(ETa) is the actual amount of water lost from the soil during field
growing conditions. The reference crop ET is related to the actual by the
equation:
(2.7)
KC is a coefficient accounting for crop maturity and water
stress under which the plant is growing. KC values vary with the crop, its
stage of growth, growing season and prevailing weather conditions. From the
equation (2.7) the actual ET is obtained from the reference. This is because
depends only on climatic factors and hence, it is easier to obtain
and then apply the crop coefficient (KC). ETa can
be obtained directly for example, by determining the moisture content (MC) of
the soil between a given time interval. However, this approach is slow and
tedious and is used only as a check on indirect methods.
Table 2.1: Length of crop growth developmental stages
for various planting periods and Climatic regions
|
Stages of Development
|
Plant date
|
Region
|
|
|
|
|
Crop characteristic
|
Initial
|
Crop Development
|
Mid- season
|
Late
|
Total
|
|
|
Banana 1st year
|
|
Stage length, days
|
120
|
90
|
120
|
60
|
390
|
March
|
Mediterranean
|
Depletion Coefficient, p
|
0.35
|
>>
|
0.35
|
0.35
|
-
|
|
|
Root Depth, m
|
0.30
|
>>
|
>>
|
0.80
|
-
|
|
|
Crop Coefficient, C
|
0.5
|
>>
|
1.1
|
1.0
|
-
|
|
|
Yield Response Factor, Ky
|
|
|
|
|
1.2- 1.35
|
|
|
Banana 2nd year
|
|
Stage length, days
|
120
|
60
|
180
|
5
|
365
|
February
|
Mediterranian
|
Depletion Coefficient, p
|
0.35
|
>>
|
0.35
|
0.35
|
-
|
|
|
Root Depth, m
|
0.30
|
>>
|
>>
|
0.8
|
-
|
|
|
Crop Coefficient, C
|
1.0
|
>>
|
1.2
|
1.1
|
-
|
|
|
Yield Response Factor, Ky
|
|
|
|
|
1.2- 1.35
|
|
|
|
Source: Allen et al., 1998
Table 2.2: Monthly Kc values of Banana for
tropical climate
Months after planting
KC
|
Crop Developmental Stage
|
|
2
|
3
|
4
|
5
|
6
|
7
|
8
|
9
|
10
|
11
|
12
|
13
|
14
|
15
|
|
0.4
|
0.45
|
0.5
|
0.6
|
.7
|
0.85
|
1.0
|
1.1
|
1.1
|
0.9
|
0.8
|
0.8
|
0.95
|
1.05
|
|
shooting
|
Harvesting
|
|
Source: Allen et al., 1998
2.3.1 Measurement of evapotranspiration
ET is not easy to measure. Specific devices and accurate
measurements of various physical parameters or the soil water balance in
Lysimeters are required to determine ET (Allen et al., 1998). The
methods are often expensive, demanding in terms of accuracy of measurement and
can only be fully exploited by well-trained research personnel. Although the
methods are inappropriate for routine measurements, they remain important for
the evaluation of ET estimates obtained by more indirect methods. The methods
used in the measurement of ET are: Energy balance and Microclimatological
methods, Soil water balance, Lysimeters, Meteorological data, Pan Evaporation.
Owing to the difficulty of obtaining accurate field measurements, ET is
commonly computed from weather data. A large number of empirical or
semi-empirical equations have been developed for assessing crop or reference
crop ET from meteorological data. Some of the methods are only valid under
specific climatic and agronomic conditions and cannot be applied under
conditions different from those under which they were originally developed.
Numerous researchers have analyzed the performance of the
various calculation methods for different location. As a result of an Expert
Consultation to compare several methods of calculation of ETo in May
1990, the FAO Penman-Montheith method is now recommended as the standard method
for the definition and computation of reference evapotranspiration,
ETo (Allen et al., 1998)
2.3.2 The Penman-Montheith equation
In 1948, Penman combined the energy balance with the mass
transfer method and derived an equation to compute the evaporation from an open
water surface from standard climatological records of sunshine, temperature,
humidity and wind speed (Allen et al.,
1998). This so-called combination method was further
developed by many researchers and extended to cropped surfaces by introducing
resistance factors.
From the original Penman-Montheith equation and the equations
of the aerodynamic and surface resistance, the FAO Penman-Montheith method to
estimate ETo is:
(2.8)
Where,
ETo reference ET [mmday-1],
Rn net radiation at the crop surface
[MJm-2day-1],
G soil heat flux density [MJm-2day -1],
T mean daily air temperature at 2 m height [oC],
U2 wind speed at 2 m height [ms-1],
es saturation vapor pressure [KPa],
ea actual vapor pressure [KPa],
(es - ea) represents the vapor pressure
deficit of the air [KPa],
ña is the mean air density at constant
pressure,
cp is the specific heat of the air,
Ä represents the slope of the saturation vapor pressure
temperature relationship, ã is the psychometric constant,
The equation uses standard climatological data of solar
radiation (sunshine hours), air temperature, humidity and wind speed. To ensure
the integrity of computations the weather data should be collected at 2 m above
the extensive surface of green grass, shading the ground and not short of
water.
2.3.3 Meteorological factors determining
evapotranspiration
The meteorological factors determining ET are weather
parameters which provide energy for vaporization and remove water vapor from
the evaporating surface (Allen et al., 1998). The principal weather
parameters to consider are:
· Solar radiation
The ET process is determined by the amount of energy
available to vaporize water. Solar radiation is the largest energy source and
is able to change large quantities of liquid water into water vapor. The
potential amount of radiation that can reach the evaporating surface is
determined by its location and time of the year. Due to differences in the
position of the sun, the potential radiation differs at various latitudes and
in different seasons. The actual solar radiation reaching the evaporating
surface depends on the turbidity of the atmosphere and the presence of clouds,
which reflect and absorb major parts of the radiation. Not all-available energy
is used to vaporize water part is used to heat up the atmosphere and soil
profile.
· Air temperature
The solar radiation absorbed by the atmosphere and heat
emitted by the earth increase the air temperature. The sensible heat of the
surrounding air transfers energy to the crop and exerts as such a controlling
influence on the rate of evaporation. In sunny warm weather, the loss of water
by ET is greater than cloudy and cool weather.
· Air humidity
While the energy supply from the sun and surrounding air is
the main driving force for the vaporization of water, the difference between
the water vapor pressure at the evapotranspiring surface and the surrounding
air is the determining factor for the vapor removal. Well-watered fields in hot
dry arid regions consume large amount of water due to the abundance of energy
and the desiccating power of the atmosphere. In humid tropical regions,
notwithstanding the high-energy input, the high humidity of the air will reduce
the ET demand. In such an environment the air is already close to saturation,
so that less additional water can be stored and hence the ET rate is lower than
in arid regions.
· Wind speed
The process of vapor removal depends largely on wind and air
turbulence, which transfer large quantities of air over the evaporating
surface. When vaporizing water, the air above the evaporating surface gradually
becomes saturated with water vapor. If this air which is the driving force for
water vapor removal is not continuously replaced with drier air ET rate
decreases.
2.4 Maximum Production
Banana cultivation is mainly geared towards the production of
fruits, for the production of beer, animal feeds and not the least the
production of fibers for textile industry (Marty, 1983). In this study, the
objective of banana cultivation is for the production of fruits. Many models to
estimate the potential production of crops have been developed. Doorenbos
et al., (1986) presented the Dewit model in 1965 as a model of
estimation of the potential production of crops. This model unfortunately has
not yet been adopted for the banana crop surely due to the complexity of this
perennial herbaceous plant. Smith et al., (1996) concluded that
agricultural production is affected by the level of water stress that will be
experienced by the crops during development. This model goes from
E
linear regression, to bring in the rate of production reduction
to arrive at a relation that
? 1 ?
? E x
Smith et al (1996) called Crop Water Yield Function?
(CWYF). The summarized equation is as follows:
(2.9)
In this equation Ya is the actual production in
tons/hectare (t/ha); Ymax is the maximum production in t/ha;
ETa is the actual evapotranspiration in mm/season; ETmax
is the maximum evapotranspiration in mm/season and Ky is the yield
response factor and has no unit. Ky describes the reduction in
relative yield due to water stress. Doorenbos et al., (1986) suggested
that for banana, Ky will have a value between 1.2 and 1.35,
represents the rate of evapotranspiration reduction, and
represents the rate of production reduction due to water
stress
The problem with this model is the determination of the
maximum production of a banana plantation with respect to only the response to
water, indifferently from other factors of production such as soil factors,
crop factors, climate and topography.
Beernaert and Bitondo (1993) in their effort to consider many
factors for the estimation of the potential production of crops presented a
model called Modèle d`évaluation des terres?. This model
considers factors such as winds, fungus or nematodes diseases, the crop species
cultivated and others, and is more reliable than other models.
They have used this model on many crops including banana. They
estimate that in ideal conditions (without limitations) the potential
production of a banana plantation ranges between 40 and 60 tons/ha.
2.5 Pressurized Irrigation Systems 2.5.1 Sprinkler
irrigation systems
Sprinkler irrigation is a versatile means of applying water to
any crop, soil, and topographic condition (Schwab et al., 1993; Fonteh
and Assoumou, 1996). Sprinkler systems can be efficient on soils and topography
that is not suitable or efficient for surface irrigation methods. In general,
systems are described according to the method of moving the lateral lines on
which various types of sprinklers are attached. Laterals may be stationary or
movable. Sprinkler systems are highly efficient but there are general concerns
about the maintenance and investment costs for these systems.
Hand-moved laterals have the lowest investment cost but the
highest labour requirement. These systems are only suitable for short growing
crops.
The side roll lateral system uses the irrigation pipe as the
axle of large diameter wheels that are spaced about 12 m apart. These laterals
are moved by a gasoline powered motor and thus require less labor than
hand-move systems. Side rolls should be used for crops that will not interfere
with the movement of the lateral or sprinkler pattern.
Centre pivots consist of radial pipelines that rotate around a
central pivot by water pressure, electric motors, or oil hydraulic motors
(Schwab et al., 1993). A variety of nozzle types, nozzle heights, and
application rates can be used in centre pivot systems. Sprinkler systems are
selected according to the field conditions for the most efficient operation.
Linear moved laterals use hardware similar to that of a centre
pivot, but move in a straight line across the field. Solid-set systems have
sprinklers that are placed over the entire field, where all or some of the
sprinklers may operate at the same time. Sprinkler heads vary greatly from
older impact heads to more modern spray heads that have an assortment of
application and placement modes (Howell, 2003).
2.5.2 Micro irrigation systems
Micro irrigation is a method for delivering slow, frequent
applications of water to the soil using a low pressure, low volume distribution
system and special flow-control outlets (Schwab et al., 1993). If
managed properly, micro irrigation can increase yields and decrease water,
fertilizer, and labor requirements. Micro irrigation includes: micro
sprinklers, drip irrigation, and subsurface drip irrigation (SDI).
Micro sprinklers, often referred to as micro sprayers or
misters, typically consist of small emitters placed on short risers above the
soil surface. Water is conveyed through the air, but travels only a short
distance before reaching the soil surface. The wetted area of emitters in these
systems is small, can be controlled fairly easily, and has different shapes to
match desired distribution patterns. The advantages of micro sprinkler
irrigation systems are the potential for controlling frost, greater flexibility
in applying water, and lower susceptibility to clogging.
Drip systems deliver water directly to the soil surface or
subsurface (SDI) and allow water to dissipate under low pressure in a
predetermined pattern. These systems are advantageous because water is applied
directly to or just above the root zone of the plant, thereby minimizing deep
percolation losses, reducing or eliminating the wetted area from which water
can evaporate, and eliminating losses associated with runoff. These systems are
also advantageous because they reduce water consumption by weeds, while
operating at a lower pressure.
Micro irrigation systems apply water on a high-frequency basis
and create near optimal soil moisture conditions for the crop. Under proper
management, micro irrigation systems save water because only the plant`s root
zone is supplied with water and little, if any, is lost to deep percolation,
consumption by non beneficial plants, or soil surface evaporation. In addition
to being highly efficient, these systems also require relatively little labour
input if designed properly. Yields of some crops have been shown to increase
under these systems because the high temporal soil water level needed to meet
transpiration requirements is maintained (Colaizzi et al., 2003).
The major disadvantages of micro irrigation systems are high
initial cost and potential for the emitters clogging. In some cases, labor
inputs may be quite high if rodents
burrow and chew system components. Proper design, operation, and
maintenance can overcome many of these issues.
2.6 Irrigation Scheduling and Management 2.6.1
Irrigation management
Irrigation management can be defined as the process of
implementation of suitable operation and maintenance in order to meet the
objectives of the concerned irrigation system and monitoring of the activities
to assure that the objectives are met.
Three implications can be drawn from the above definition.
First of all, that irrigation management is not a routine job. The management
decisions have to be made with great care, as they have to match with the
operation and maintenance objectives. Secondly, even though the overall goal
may be the same, objectives vary from system to system, hence management
decisions have to take account of these inter system differences. Thirdly, that
monitoring is an integral part of management thus management decisions have to
be continuously refined according to the feedback obtained from monitoring and
evaluation.
Irrigation Management is one of the major challenges for the
irrigation professionals. It is important as it decides the benefit derived
from the irrigation system.
2.6.2 Irrigation scheduling
Irrigation performance can be improved either by means of
developing new application systems (drip, sprinkler, etc.) or by a more
accurate irrigation scheduling. For any crop, schedule implies the
determination of time and volume of water application to meet a specified
management objective.
Jensen (1981) defines irrigation scheduling as a planning and
decision-making activity that the farm manager or operator of an irrigation
farm is involved in before and during most of the growing season for each crop
that is grown. It could also be defined as the use of water management
strategies to prevent over application of water while minimizing yield loss due
to water shortage or drought stress. He further indicated four types of data
needed for irrigation decision making:
1) Current level and expected change in available soil water for
each field over the next 5 to 10 days.
2) Current estimates of the probable latest date of the next
irrigation on each field to avoid adverse effects of plant water stress.
3) The amount of water that should be applied to each field,
which will achieve high irrigation efficiency.
4) Some indication of the adverse effects of irrigation a few
days early or late.
Irrigation scheduling requires a particular attention because
of its influence on irrigation efficiency and its consequences on the
environment. The water holding capacity of the soil and the suction that the
cultivated crop can develop on the soil water are good guides for irrigation
scheduling. The techniques used currently for irrigation scheduling are
diverse. Relative to the equipments that are used for these techniques, they
can be sophisticated or very simple. Methods based on direct measurements of
plant water status have always attracted the attention of irrigation research
as a tool for irrigation timing, but getting accurate and representative data
for these parameters has always been very difficult (Cremona et al.,
2000). Based on the soil-plant-water relations, Kramer (1983) suggested
that the determination of the time and quantity of water to supply by
irrigation could be obtained by either one of the three fundamental methods
namely:
· Determination of soil moisture
· Estimation of the water used by plants from climatic
data
· Measure of water stress that has affected the crop
Irrigation scheduling to satisfy the water requirement of
plants must conform to the hydrology of the milieu and to the objectives set by
the irrigation practice. Njila (1999), stated that most irrigation managers in
Cameroon prefer to irrigate their crops following a pre-established
calendar.
Whatever the context, Fonteh & Assoumou (1996), Tron et
al. (2000), present two fundamental questions that need to be answered in
any irrigation scheduling program:
- When to irrigate?
- How much water to apply?
Smith et al, (1996) classified scheduling options into
two different categories as follows:
a) Timing options - related to when irrigation is to be
applied:
1) Each irrigation defined by user; this type is used to
evaluate irrigation practices and to simulate any alternative irrigation
schedule.
2) Irrigation at critical depletion (100 % depletion of
readily available soil moisture). Resulting in minimum irrigations, but
irregular and therefore unpractical irrigation intervals.
3) Irrigation below or above critical depletion (% depletion
of readily available soil moisture). Useful to set a safety level above
critical soil moisture or allow a critical stress level.
4) Irrigation at fixed intervals per stage, suitable in
particular in a gravity system with rotational water distribution, may result
in some over-irrigation in the initial stages and under-irrigation in the peak
season.
5) Irrigation at given crop evapotranspiration reduction
(%).
6) Irrigation at given yield reduction (%).
7) No irrigation, only rainfall.
b) Application options - how much water is to be given
per irrigation turn:
1) Each irrigation depth is defined by the user, as determined
from field or simulated data.
2) Refill soil to field capacity, to bring soil moisture
content back to field capacity, thus equal to the depleted soil moisture in the
root zone, as the depletion in the root zone will normally vary over the
growing season with changing root depth and allowable depletion levels.
3) Refill below or above field capacity. Useful to allow for
leaching for salinity control
(above field capacity) or to accommodate possible rainfall (below
field capacity).
Irrigation scheduling schemes should take into account factors
such as the soil properties that affect soil moisture-holding capacity. James
et al. (1982) for example, reported that irrigation scheduling with a
soil of low water-holding capacity would have to be more frequent with smaller
amounts applied each time for best efficiency.
2.6.3 Importance of irrigation scheduling
Some irrigation water is stored in the soil to be removed by
crops and some is lost by evaporation, runoff, or seepage. The amount of water
lost through these processes is affected by irrigation system design and
irrigation management. Prudent scheduling minimizes runoff and percolation
losses, which in turn usually maximizes irrigation efficiency by reducing
energy and water use.
Energy can thus be saved by no longer pumping water that was
previously being wasted. When water supplies and irrigation equipment are
adequate, irrigators tend to over irrigate, believing that applying more water
will increase crop yields. Instead, over irrigation can reduce yields because
the excess soil moisture often results in plant disease, nutrient leaching, and
reduced pesticide effectiveness. In addition, water and energy are wasted.
The quantity of water pumped can often be reduced without
reducing yield. Studies have shown that irrigation scheduling using water
balance methods can save 15 to 35 percent of the water normally pumped without
reducing yield (Evans et al., 1996). Maximum yield usually does not
equate to maximum profit. The optimum economic yield is less than the maximum
potential yield. Irrigation scheduling tips presented in popular farm magazines
too often aim at achieving maximum yield with too little emphasis on water and
energy use efficiencies. An optimum irrigation schedule maximizes profit and
optimizes water and energy use.
2.7 Geographic Information Systems
A geographic information system (GIS), or geographical
information system is a system which captures, stores, analyzes, manages, and
presents data that is linked to location (Chang, 2007). GIS provides a means of
measuring spatial and attribute data into a computerized database system,
thereby allowing input, storage, retrieval and analysis of geographically
referenced data (Heywood et al., 2006). It is therefore a system of
computer hardware, software, and procedures designed to support the capture,
management, manipulation, analysis, modeling, and display of spatially
referenced data for solving complex planning and management problems. In the
strictest sense, the term describes any information system that integrates
stores, edits, analyzes, shares, and displays geographic
information. In a more generic sense, GIS applications are
tools that allow users to create interactive queries (user created searches),
analyze spatial information, edit data, maps, and present the results of all
these operations. Analyzing large amount of data is a necessity for management
of irrigation projects. Data must be collected, stored and interrelated with
each other in such a way that the data are readily accessible (Dayyani et
al., 2003). The cartographic and data overlaying capability of GIS coupled
with its dynamic linking ability to models plays a vital role in water
management. In addition, its ability of writing scripts gives the decision
makers this power to produce the necessary outputs the way they need them.
GIS technology can be used for water resource management,
asset management, archaeology, environmental impact assessment, urban planning,
cartography, criminology, geographic history, marketing, logistics, scientific
investigations, prospectivity mapping, and other purposes. For irrigation
management adequate and updated information regarding the irrigation system is
needed, thus GIS tool for irrigation management provides information
interactively for decision making process. GIS have the capability of improving
water management techniques as well as decision-making (Taylor, 2005). GIS have
thus, taken a central role in analyzing, modeling, and managing a wide range of
water resource information. System GIS can analyze spatial interactions between
static and dynamic entities.
1. Spatial data management
2. Interactive visualization
3. Spatial analysis
4. Customization and decision-making support.
The importance of spatial geographic components in on-farm
irrigation system performance imposes the involvement of the capabilities to be
able to store, aggregate, manipulate, analyze and visualize a huge quantity of
data. In the recent last ten years, to this purpose, the use of GIS has been
greatly diffused. These systems, if combined to appropriate simulation models
could support the decisions of designers and/or managers (Hoogenboom et
al., 1991).
A GIS is characterized by a unique ability of the user to
overlay spatial layers, each, representing one or more physical and/or
functional characteristics of the studied
phenomenon. Each layer is related to a table, representing the
database. Using appropriate models, it is then possible to actively elaborate
the information and to present results under tabular and/or maps form.
There have been several applications of GIS in irrigation and
drainage systems around the world. Sarangi et al., (2001) used GIS in
development of input data set for a conceptual small watershed runoff
generation model. In addition, they used ARC/INFO for canal system within the
project area of Patna Canal and distributaries of Sone command area in India.
Amor et al., (2002) combined GIS with a crop growth model to estimate
the water productivity in time and space in the Philippines. Three products,
rice, corn and peanut were modeled in their research. They analyzed the water
limitation for each crop in different seasons and determined the productivity
potential in the region. In Iran, application of GIS dates as far back as the
90's in diverse fields of water sciences such as hydrology, flood control,
water erosion, and groundwater management. Daneshkar et al. (2000)
used GIS and Modflow for simulation of Ab-Barik groundwater plain. Alvankar
et al. (2000) applied GIS in watershed characterization of the Latvian
dam watershed.
2.7.1 Data acquisition and representation
GIS data represents real world objects (roads, land use,
elevation) with digital data. Real world objects can be divided into two
abstractions: discrete objects (a house) and continuous fields (rain fall
amount or elevation). There are two broad methods used to store data in a GIS
for both abstractions: Raster and Vector.
a) Raster data
A raster data type is, in essence, any type of digital image
represented in grids. While a digital image is concerned with the output as
representation of reality, in a photograph or art transferred to computer, the
raster data type will reflect an abstraction of reality. Aerial photos are one
commonly used form of raster data, with only one purpose, to display a detailed
image on a map or for the purposes of digitization. Other raster data sets will
contain information regarding elevation or reflectance of a particular
wavelength of light.
Raster data type consists of rows and columns of cells, with
each cell storing a single value. Raster data can be images (raster images)
with each pixel (or cell) containing a color value. Additional values recorded
for each cell may be a discrete value, such as land
use, a continuous value, such as temperature, or a null value
if no data is available. While a raster cell stores a single value, it can be
extended by using raster bands to represent RGB (red, green, blue) colors,
color maps (a mapping between a thematic code and RGB value), or an extended
attribute table with one row for each unique cell value. The resolution of the
raster data set is its cell width in ground units. Raster data is stored in
various formats; from a standard file-based structure of Tagged Image File
(TIF), Joint Photographic Experts Group (JPEG), etc. to binary large object
(BLOB) data stored directly in a relational database management system (RDBMS)
similar to other vector-based feature classes. Database storage, when properly
indexed, allows for quicker retrieval of the raster data but can require
storage of millions of significantly-sized records.
b) Vector data
In a GIS, geographical features are often expressed as
vectors, by considering those features as geometrical shapes. Different
geographical features are expressed by different types of geometry:
· Points
Zero-dimensional points are used for geographical features
that can best be expressed by a single point reference; in other words, simple
location. Points can also be used to represent areas when displayed at a small
scale. For example, cities on a map of the world would be represented by points
rather than polygons. No measurements are possible with point features.
· Lines or polylines
One-dimensional lines or polylines are used for linear
features such as rivers, roads, railroads, trails, and topographic lines.
Again, as with point features, linear features displayed at a small scale will
be represented as linear features rather than as a polygon. Line features can
measure distance.
· Polygons
Two-dimensional polygons are used for geographical features
that cover a particular area of the earth's surface. Such features may include
lakes, park boundaries, buildings, city boundaries, or land uses. Polygons
convey the most amount of information of the file types. Polygon features can
measure perimeter and area.
Each of these geometries is linked to a row in a database
that describes their attributes. For example, a database that describes water
resource may contain its depth, water quality, pollution level. This
information can be used to make a map to describe a particular attribute of the
dataset. For example, water resources could be colored depending on level of
pollution. Different geometries can also be compared.
Vector features can be made to respect spatial integrity
through the application of topology rules such as 'polygons must not overlap'.
Vector data can also be used to represent continuously varying phenomena.
Contour lines and triangulated irregular networks (TIN) are used to represent
elevation or other continuously changing values. TINs record values at point
locations, which are connected by lines to form an irregular mesh of triangles.
The faces of the triangles represent the terrain surface.
2.7.2 Advantages and disadvantages of vector and
raster data
There are advantages and disadvantages to using a raster or
vector data model to represent reality.
Raster datasets record a value for all points in the area
covered which may require more storage space than representing data in a vector
format that can store data only where needed. Raster data also allows easy
implementation of overlay operations, which are more difficult with vector
data.
Vector data can be displayed as vector graphics used on
traditional maps, whereas raster data will appear as an image that, depending
on the resolution of the raster file, may have a blocky appearance for object
boundaries.
Vector data can be easier to register, scale, and re-project.
This can simplify combining vector layers from different sources. Vector data
is more compatible with relational database environments. They can be part of a
relational table as a normal column and processed using a multitude of
operators.
The file size for vector data is usually much smaller for
storage and sharing than raster data. Image of raster data can be 10 to 100
times larger than vector data depending on the resolution. Another advantage of
vector data is that it is easy to update and maintain.
2.7.3 Steps used for the putting in place of a GIS
project
In a typical GIS project, there is need to identify the
objectives of the project, create a database specifically for the project and
containing all information for the resolution of the problem, then, we need to
use GIS functions to create an analytical model that will be able to solve the
problem and lastly present the results of the analysis. ESRI (1996), define
four basic steps in the development of a GIS project.
Step 1: Identification of objectives
The first step of the procedure consists in identifying the
objective of the analysis. The following questions need to be taken into
consideration in order to define the objectives:
· What is the problem to be solved? How is the problem
being solved actually? Are there any more solutions with the aid of GIS?
· What are the expected results: reports, study maps,
presentation maps?
· To whom are the results destined: management,
technicians?
· Are the data going to be used for other goals? What are
the conditions in this case?
This is an important step as the answers to the latter questions
define the objectives of the project as well as the methods to be used in
putting in place the system.
Step 2: Creation of a database
The second step consists of creating a database for the
project. The creation of this database is a three stage procedure. The stages
help in the conception of the database, to automate and to assemble the data
and then manage this data. The design of a database comprises:
· The identification of the necessary spatial data with
respect to the need in analysis, definition of the different attributes
required by the various entities, definition of the boundaries of the study
area and the choice of the coordinate system to be used,
· Automating access to the data which comprises the
digitization or the conversion of data from other systems and formats to a
useable format, as well as the verification of the data and correction for
errors, and
· Management of the data which comprises the verification
of the coordinate system and union of two adjacent layers.
Creation of a database for the project is of prime importance
and usually takes a greater part of the time required for a GIS project. The
completeness and precision of the data used in analyses will equally determine
the precision of the results. This explains why this study is centered towards
the creation of a database for the irrigation system of the PHP group.
Step 3: Data analysis
This third step consists of analyzing the data obtained.
Analysis of GIS moves from simple realization of maps to the creation of
complex spatial models. A model is the representation of reality used to
simulate a given process, predict a given result or analyze a given problem. A
spatial model applies one or more of these three categories of functions of
GIS:
· Geometric modeling - calculation of distances, areas and
perimeters, creation of buffer zones,
· Coincidence modeling - superposition of various data to
find their locations or the coinciding values,
· Contiguity modeling- allocation, search for routes and
cutting into sectors.
With the use of GIS it is possible to carry out rapid
analysis which would have been extremely long or even impossible to do
manually. Different scenarios are being created by changing the method or a
parameter of an event and executing the analysis to obtain various results.
Step 4: Presentation of Results
This is the final stage of a GIS project. In most cases, GIS
results are better shown on maps. Diagrams and data reports are equally other
methods of presenting results. These diagrams and reports could be printed
separately, incorporated into created files or placed on maps.
2.8 Databases
A Database is a structured collection of data that is managed
to meet the needs of a community of users (Chang, 2007). The structure is
achieved by organizing the data according to a database model. The model in
most common use today is the relational model. Other models such as the
hierarchical model and the network model use a more
explicit representation of relationships. A computer database
relies upon software to organize the storage of data. This is defined as
database management system (DBMS). Some terms whose meaning is required to
understand databases are (Chang, 2007):
· File
A file is an ordered arrangement of records in which each
record is stored in a unique identifiable location. The sequence of the record
is then the means by which the record will be located. In most computer
systems, the sequence of records is either alphabetic or numeric based on field
common to all records such as name or number.
· Records
A record or tuple is a complete set of related fields. For
example, Table 2.3 shows a set of related fields, which is a record. In other
words, if this were to be a part of a table then we would call it a row of
data. Therefore, a row of data is also a record.
Table 2.3: Set of related fields in an irrigation system
which form a record
Id_plot #laterals #sprinklers Flow rate
|
|
Field Record
|
Nyombe 255 445 250
|
|
|
· Field
A field is a property or a characteristic that holds some
piece of information about an entity. Also, it is a category of information
within a set of records. For example, the first names, or address or phone
numbers of people listed in address book.
· Relations
In the relational data model, the data in a database is
organized in relations. A relation is synonymous with a =table`. A table
consists of columns and rows, which are referred as field and records in DBMS
terms, and attributes and tuples in Relational DBMS terms. A comparison between
DBMS and RDBMS terms is given in Table 2.4.
· Attributes
An attribute is a property or characteristics that hold some
information about an entity. An irrigation network for example starts from a
pumping station which has attributes such as a name, given number of pumps and
engines etc.
Table 2.4: Comparing DBMS and Relational DBMS (RDBMS)
terms
Common Term DBMS Terminology RDBMS
Terminology
Database Table Database
Table Table Relation
Column Field Attribute
Row Record Tuple
2.8.1 Database management systems
A Database Management System (DBMS) is a set of computer
programs that control the creation, maintenance, and the use of the database of
an organization and its end users (Codd, 1970). It controls the organization,
storage, management, and retrieval of data in a database. DBMS are categorized
according to their data structures or types, sometime DBMS is also known as
database manager. It is a set of prewritten programs that are used to store,
update and retrieve a database. A DBMS includes:
- A modeling language to define the scheme of each database
hosted in the DBMS, according to the DBMS data model.
- Data structures (fields, records, files and objects)
optimized to deal with very large amounts of data stored on a permanent data
storage device (which implies relatively slow access compared to volatile main
memory).
- A database query language and report writer to allow users
to interactively interrogate the database, analyze its data and update it
according to the users privileges on data.
The four most common types of organizations are the
hierarchical, network, relational and object models. Inverted lists and other
methods are also used. A given database management system may provide one or
more of the four models. The optimal structure depends on the natural
organization of the application's data, and on the application's requirements
(which include transaction rate (speed), reliability, maintainability,
scalability, and cost).
Some DBMS software include Microsoft Access, Oracle DB2,
Sybase Adaptive Server Enterprise, FileMaker Firebird, INGRES, Informix,
Microsoft SQL Server, Microsoft
Visual FoxPro, MySQL, PostgreSQL, Progress, SQLite, Teradata,
CSQL, OpenLink Virtuoso.
Some benefits obtained from the use of DBMS could include:
· Improved strategic use of corporate data,
· Reduced complexity of the organization`s information
systems environment,
· Reduced data redundancy and inconsistency,
· Enhanced data integrity,
· Application-data independence,
· Improved security,
· Improved flexibility of information systems,
· Increased access and availability of data and
information,
· Logical & physical data independence,
· Concurrent access anomalies,
· Facilitate atomicity problem,
· Provides central control on the system through database
applications.
2.8.2 Relational databases
A short definition of a RDBMS may be a DBMS in which data is
stored in the form of tables and the relationship among the data is also stored
in the form of tables (Codd, 1970). Data items are organized as a set of
formally-described tables from which data can be accessed or reassembled in
many different ways without having to reorganize the database tables.
The relational model is the most commonly used today. It is
used by mainframe, midrange and microcomputer systems. It uses two-dimensional
rows and columns to store data. The tables of records can be connected by
common key values. There are 3 relationship types:
One-to-one (1:1) - Each record in Table A
can have only one matching record in Table B and each record in Table B can be
related to only one record in Table A as shown in figure 2.2. This type of
relationship is not frequently used in database systems, but it can be very
useful way to link two tables together. However, the information related in
this way could be in one table. They may be used to divide a table with many
fields in order to isolate part
of a table for security reasons, or to store information that
applies only to a subset of the main table, or for efficient use of space. A
one-to-one relationship is created if both of the related fields are primary
keys or have unique indexes.
Table A Table B
1
1
irrigation plot
Id irrigation Plot id plot
valve id_irrigation_system number of sprinklers plot name
Plot valve
Id plot valve Longitude latitude Altitude
Figure 2.2: One-to-one relationship of
databases
This shows that, one irrigation plot could have one and only
one valve for the control of the flow of water in the plot.
One-to-many (1:M) - It is the most common
type of relationship and it is used to relate one record from the 'primary'
table with many records in the 'related' table. In a one-tomany relationship, a
record ('parent') in Table B can have many matching records ('children') in
Table A, but a record ('child') in Table A has only one matching record
('parent') in Table B as shown in Figure 2.3. This kind of relationship is
created if only one of the related fields is a primary key or has a unique
index.
Table A Table B
Production plot
n 1
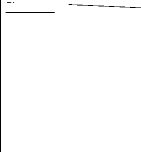
Plot name Id_production sector Soil type
Crop variety Planting date Agronomic state Spatial arrangement
Irrigation plots
Total surface area slope
Name_station
Production sector
Id_production sector Surface area
Id_plantation
|
|
Figure 2.3: One-to-many relation of
databases
From Figure 2.3 it could be seen that, one production sector
could be related to many production plots, but one production plot belongs to
one and only one production sector.
Many-to-many (M:M) - is used to relate many
records in the Table A with many records in the Table B. A record ('parent') in
Table A can have many matching records ('children') in Table B, and a record
('child') in Table B can have many matching records ('parents') in Table A. By
breaking it into two one-to-many relationships and creating a new
(junction/link) table to stand between the two existing tables will enable
correct and appropriate relationship setting. A many-to-many relationship is
really two one-to-many relationships with a junction/link table.
A production plot for example could have many soil types and
this soil type could be found in many other production plots around the
plantation.
CHAPTER III MATERIALS AND METHODS
3.1 Description of the Study Area and Experimental
Site 3.1.1 Geographical Location
Njombé covers a surface area of about 260
km2 and is located between latitudes 4°30`N and 4°40`N and
longitudes 9°30`E and 9°45`E. The average altitude of the area is 140
m. Njombé is bounded:
· to the North by Penja,
· to the South by Mbanga,
· to the West by Tombel,
· and to the East by the Nkam.
Figure 3.1 provides the geographical location of Njombé
while Figure 3.2 shows the area cultivated.
PHP group cultivates bananas and pineapples on a surface area
of about 3 500 ha and is divided into plantations, with one of them being the
Njombé plantation. These plantations in Njombé are an integral
part of the zone of production of PHP. In 2004, the cultivated land was about
2250 ha (Boa, 2005). The plantations are further divided into sectors and the
sectors into production plots.
3.1.2 Relief
Njombé has a relatively flat topography, made up of
plateaus and some eroded undulating landscape. One could notice the effects of
an old volcanic activity in the area (Tchiadje 1995).
3.1.3 Hydrology
The area has numerous water bodies and most of them are
exploited by the companies present in the area such as PHP, Tangui, SPM and by
the peasants for their day-to day activities. The principal water bodies are:
the Moungo, Moulinkam, Moumbé, Bwale, Mbomé, and Ngomba.
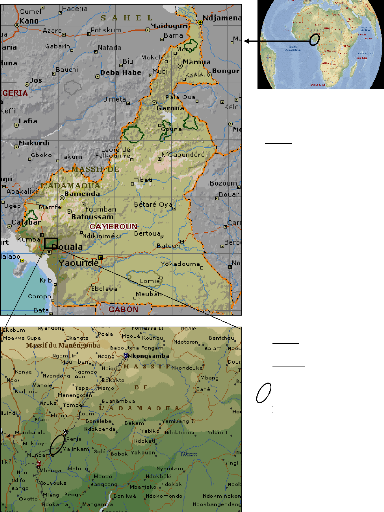
Scale :
1/20000000 Source : Encarta 2008
Scale :
1/1000000 Source : Encarta 2008
PHP plantations
Figure 3.1: Geographical location of
Njombé
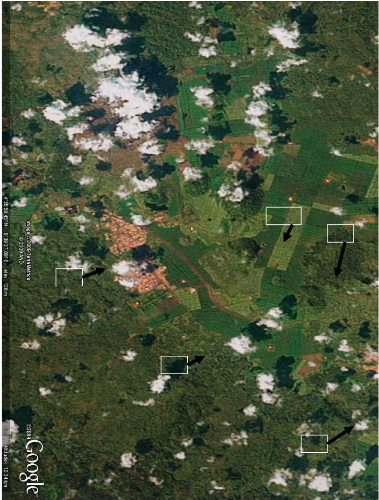
N
R
B
KEY
C
C: Hill
P
B: PHP cultivation
plots
P: Penja town
R: National road n°2
N: Njombe town Scale: 1/14000 Source: Google Earth version 5
Figure 3.2: Aerial view of PHP cultivation areas in the
Njombé Plantations
3.1.5 Vegetation
Njombé is situated in the Guineo - Congolese floristic
region, in the dense humid forest sector of the biafran forest. This forest
constitutes plants of the Cesalpiniaceae family. Some of the most
characteristic trees species found in the area are: Bidou (Saccaglottis
gabonensis) and Azobe (Lophira alata) (Van de
Pol et al, 2005). Due to the high fertility of soils in this area, there has
been serious deforestation for the setting up of either large plantations or
small farms or for the building of houses due to rapid urbanization.
Nevertheless, one could still notice some forest reserves rich in timber and
other forest species.
3.1.6 Climate
The climate of the area is equatorial with a long rainy season
which runs from March to November and a short dry season which runs from
November to February. It is a hot and humid climate with temperatures of
25-30°C and an average relative humidity of 80%. The cumulative average
annual potential evapotranspiration equals 1 055.6mm (an average weekly ETo of
20.3mm) while the annual rainfall averages about 2550mm (Thome, 2007). Table
3.1 shows the average annual precipitation of Njombé for a 5 year period
(2004-2008).
Table 3.1: Average annual precipitation of Njombé
(2004-2008)
Year
|
Precipitation (mm)
|
2004
|
2320
|
2005
|
2385
|
2006
|
2445
|
2007
|
2960
|
2008
|
2636
|
Mean
|
2550
|
Source: Climatic data from PHP meteorological
stations
Figure 3.3 shows the ombrothermic graph for Njombé area
for the year 2008. The graph shows the rainfall amounts and evapotranspiration
for the area for each week of the year and could be used to determine the start
and the end of the rainy and dry seasons. The peak of the rainfall lies between
the 26th and 29th month and almost zero from the
47th week to the 8th week of the year.
Figure 3.3: Monthly rainfall histogram
for Njombé in 2008 3.1.7 Soils
The soils in the area are mostly ferralitic in nature. These
are shallow soils with a clayey-sand granulometry. The pH of these soils varies
between 4.5 and 6.5. They are thus very fertile soils, rich in mineral content.
According to Defo and Marie, (1998), the soils in the banana areas of the
Moungo which are of volcanic origin have undergone several studies. These
studies have helped to distinguish with respect to the level of weathering of
the bedding rock. We could thus distinguish three types of soils:
1) Recent soils: These are andosols dominated by allophones.
These soils are characterized by a high porosity, a llight silty texture, a
fragile granular structure and an important number of stones.
2)
Soils dominated by Halloysites: These soils have a silty-clay
texture with a
ETP (m)
stable granular structure.
m
3) Highly weathered soils: These soils are dominated by
halloysites and kaolinite. They are thus soils with high clay content and thus
possess drainage problems.
3.2 Description of the Irrigation System at the PHP
Group
4 0 16 9 2 2 28 3 3 40 43
The irrigation system at the PHP Group, Njombé is divided
into three main parts; the pumping stations, the conduits (pipes), and the
distribution network. To develop the
database for the system, a clear knowledge of how the system
looks like and how it functions will be of great necessity.
3.2.1 Pumping station
The entire network is supplied through five pumping stations
(Koumbe 1, Koumbe 2, Koumbe 3, Trou Lac Dia-Dia and Maya) and use 21 pumps for
the lifting of water; 16 of the pumps are situated at the main station at
Koumbe and 5 others situated at Trou Lac, Dia-Dia and Maya. The engine pumps
used have the following characteristics:
Engine Type: 12 cylinder engine
Engine Power: 337 hp
Pump Type: Centrifugal with 5 wheels of diameters 330 mm rotating
at 1800 rpm Pump Flow Rate: 240 m3/h
Total Head: 240 m
Operating Pressures: 24 bars (Koumbe) and 13-17 bars (others)
Water is pumped from the rivers Moumbe, Mboko and Maya as well as
the Dia Dia Lake and Well (about 50 m deep).
3.2.2 The main line (Pipes)
Water is transported from the pumping stations to the
plantation via steel or PVC pipes with diameters between 200 mm and 700 mm.
These pipes are either buried, placed on the surface or have become exposed to
the surface due to erosion. Interconnection between the pipes gives a looped
network such that in case of malfunctioning of one of the pumping stations, the
others could be used for pumping. During the irrigation season, each pipe
operates individually, hence, forming a ramified network. These ramified
networks cover a total distance of about 55 km and each is equipped with a
given set of structures and equipments, which facilitate the use and management
of these networks. Figure 3.4 shows these main conduit and the pumping stations
from which they obtain water.
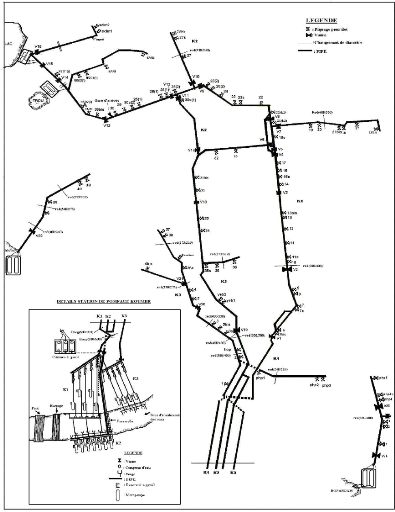
Source: Irrigation department PHP
Figure 3.4: Main lines at the PHP group
3.2.3 Distribution network
This is the set of equipment and structures which transport water
from the main hydrant to the level of the sprinklers. It is made up of:
- Secondary conduits in galvanized steel or polyethylene and with
diameters of 120 mm, 150 mm, or 160 mm,
- Tertiary conduits or antenna with diameters of 120 mm,
- Quaternary conduits with diameters of 40 mm or 50 mm,
- Risers and sprinklers.
This network is characterized by two main irrigation systems;
overhead and Undertree:
a) Overhead irrigation
In this type of irrigation, the sprinklers are situated above
the banana plants. This mode could either be by total coverage with big guns or
by integral coverage with sprinklers.
· Total coverage with big guns
It is the most ancient system used by the Group and usually
gives the ground base for the installation of other systems. It occupies a
surface area of about 410.20 ha. The big guns used are characterized by a flow
rate of 60 m3/h, an operating pressure of 5.5 bars, a horizontal
throw of 54.4m, coverage of 0.5 ha/canon, and an irrigation depth of 10mm/h.
the number of big guns per irrigation plot is between 60-100 big guns, this
gives a spacing of 72 or 78 m in the line and between 66 or 73 m between lines
(72 m*66 m or 78 m*73 m). The advantage of using big guns is the ease of follow
up of irrigation but a great disadvantage lies in the high energy consumption
during pumping.
· Integral coverage with sprinklers
(21*21)
It is characterized by single or twin nozzle sprinklers with
the following features: a flow rate of 1.4 m3/h for single nozzle
sprinklers or 1.9 m3/h for the twin nozzle sprinklers, an operating
pressure of 4 bars, and an irrigation depth of 3.36 mm/h. Evaporation and wind
are the principal setbacks to this system. Compared to big guns however, this
system consumes less energy. It occupies a surface area of 193.46 ha.
b) Undertree irrigation
Two systems are used in this Group; sprinklers with integral
coverage (12*11 m) and the micro jets system.
· Integral coverage
This system is made up of twin nozzle sprinkler heads. It is
a low pressure system (2.5 bars). The nominal flow rate is 0.42 m3/h
with a horizontal throw of 9.5 m per sprinkler. This system is highly prone to
theft and difficult to monitor during irrigation because of the high density
per irrigation plot (200-250 sprinklers/plot). It occupies a surface area of
1250.25 ha.
· Micro Jets
Used mostly in the cluster and twin line spatial arrangement.
The sprinkler heads used are of the type RONDO and RAINBIRD and are
auto-regulated (flow rate does not change with variation in pressure). The
characteristics of these micro sprinklers are; a flow rate of 30 l/h, an
operating pressure of 1.5 and 3.5 bars for horizontal throws of 1.8 and 2.0
bars respectively. Despite the advantage of low energy consumption, it requires
special attention in terms of maintenance due to constant clogging of the
nozzles especially those of the pressure regulators which are very small. It
occupies a surface area of about 1750 ha.
3.3 Development of the Database for the Irrigation
System
The database was developed using Microsoft Access 2003 which
is a relational database management system (RDBMS). The model thus developed
under this software helped us to relate the various aspects of the system and
led to the development of a conceptual and physical model of data. Figure 3.2
shows the architecture of the GIS database
The procedure for the development of the database within MS
Access 2003 included:
· The review of existing data
· The identification of entities and their attributes
· The creation of tables, primary and foreign keys
· The definition of relationships
· Creation of data entry and retrieval forms
· Creation of queries
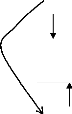
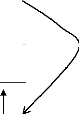
Irrigation Service

GIS DATABASE ON THE IRRIGATION SYSTEM
Crop Water Needs Irrigation
Irrigation System



Scheduling Options
Figure 3.5: Architecture of the GIS database 3.3.1 Data
review
Data was reviewed to identify entities, attributes and
facilitate the classification and coding of data. At the start of the study,
most of the data for the PHP irrigation system had been entered into MS excel
2003 spreadsheets. These excel spreadsheets were then transformed into MS
access tables. This decision was made to avoid the re-entry of data as far as
possible. The excel files where then exported to obtain MS access tables.
However, the data on the final table required some cleaning. This was preferred
to a re-entry of the data into MS access because of the time needed to re-enter
data.
3.3.2 Entity and attribute identification
Entities were identified and attributes determined. The
objective was to create normalized, non-redundant table structures. Several
entities regarding to the irrigation system have been identified with respect
to their function in the system. Thus, we started from the pumping station up
to the crop in order to determine the various components which will constitute
entities for the irrigation system database.
3.3.3 Table and key creation
On the basis of the entities determined tables were created.
A primary key was established for each table. The primary keys were defined as
Autonumber? field types to facilitate data entry and to avoid doubles in the
data. Tables for the production plots, irrigation plots, climatic data, soil
data, pumping stations, pipes, sprinklers etc have been created. Four principal
methods exist in MS access for the creation of tables.
· Design Mode
This method was used most often as it enabled us to name
tables and organize the structure of each table. This method has been used for
tables which did not exist in other formats.
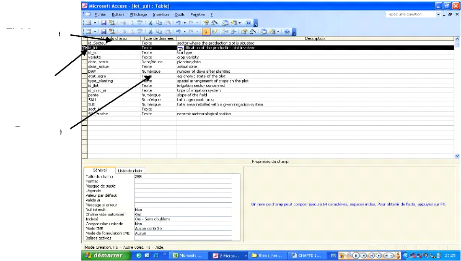
Field name
Primary key
Data type
Figure 3.6: Creation of table in design mode under MS
access
· Table assistant mode
Here, access proposes different models of pre-established
tables to choose from and create the desired table. The table was then
personalized in order for it to suit the conditions required.
· Import table mode
This method has been used for the design of most of the tables
that are being presented in this database. The rationale for this was the fact
that most of the data concerning the irrigation system has already been stored
either as texts or in MS excels spreadsheets. This made it easy for us to
import the tables to our database and then define the contents of each field to
suit the requirements of our database under the design mode by modifying the
specifications of the various fields involved.
· Attach table mode
This method has been used for files that need to remain in
their original database such as excel files on which calculations have been
done. Hence the link between these files and the attached table in MS access
remains and any modification on the file immediately leads to a modification in
the attached table. In the calculation of the crop water requirements, the
excel spreadsheet on which all the formulas were entered was exported to the
database.
3.3.4 Definition of relationships and referential
integrity
From the conceptual model of the irrigation system developed
we can easily recognize how the different entities of the irrigation system
could be related. Most of the relationships retained at the level of the
physical model were many-to-one relationships as seen in Figure 3.3. Where we
had relationships of the type many to many, we had to breakdown the
relationship into two one-to-many relationships. This was done by creating a
table between the relationships. For the relationship to be correct, there must
be fields in the two tables to link them, even if they have different names,
they should contain the same data. Each table has a primary key which is
responsible for assuring that there exists some referential integrity between
the two tables being linked together. The two tables now become related and
this helps easy access and storage of data regarding the irrigation system.
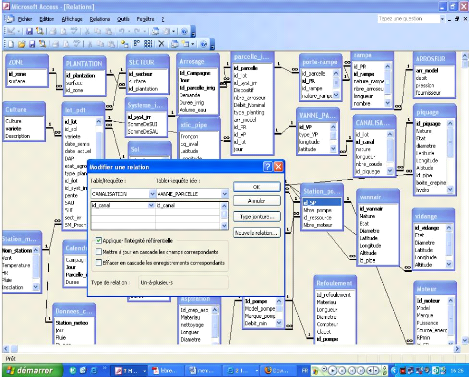
Figure 3.7: Definition of relationships
in the physical data model 3.3.5 Creation of data entry and
retrieval forms
The procedure for the creation of forms under MS access 2003 has
been used for the creation of forms for the irrigation system. This constituted
the following procedure:
1) In the Navigation pane, the tables or queries that were
needed for the form were selected to use as the form record source.
2) Then we chose Create> More> Forms> Form Wizard.
Access displays the first Form Wizard dialog box.
3) For each field we wanted to include in the form, we
clicked on the field in the Available Fields list and clicked on the >
button. (To select all the fields, we clicked on the >> button.)
4) The next Form Wizard dialog box permitted us to choose the
layout of the fields. Four choices are available for this:
· Columnar--the fields are arranged in
columns, and only one record is shown at a time.
· Tabular--the fields are arranged in a
table, with the field names at the top and the records in rows.
· Datasheet--the fields are arranged in a
datasheet layout.
· Justified--the fields are arranged
across and down the form with the field names above their respective
controls.
5) The next wizard dialog box enabled us to select one of the
predefined AutoFormat styles. Click the style you want to use and then click
Next.
6) The fourth and last wizard dialog box modifies the name of
the form. A suggestion is already in place in the What Title Do You Want for
Your Form?? Text box; it is based on the name of the underlying table or query,
but the name was entered so that it doesn`t conflict with an existing form.
Figure 3.4 shows a form under construction in MS access
environment.
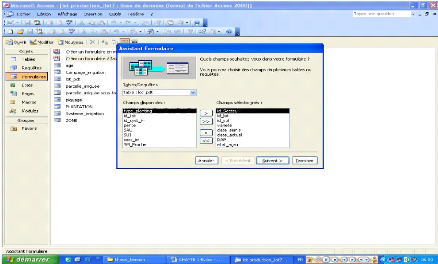
Figure 3.8: Selecting fields to be included in the
production plot form under the form assistant mode
3.4 Development of thematic layers for the GIS
The GIS was developed using MapInfo software and AUTOCAD, a
computer aided drawing (CAD) software. This type of system gives the
possibility of combining methods of simulation of spatial data and the
presentation of the results in a graphical manner that will be adapted to the
irrigation system of the PHP group. The global architecture of the system will
comprise three modules:
· Data entry and update module;
· Water balance module;
· Simulation module;
The thematic layers of the GIS were developed by reviewing the
type of data that needed to be entered in the database to meet the specific
objectives of the GIS project.
A land use map was developed for the plantation and
constituted the main thematic layer for the GIS. The plantation borders,
sector, and production plots where been represented. The procedure for creating
the land use map with the GPS involved collecting waypoints and extracting the
data to a computer. This was done through the following procedure:
1. Creating a folder on the hard drive C\MAPDATA for the data
related to the map project. This folder was not stored on the desktop as the
files are too large. A permanent folder (not to be moved or renamed) was
created, in which the data will remain, as when the maps are saved they
reference a pathway to the original data folder.
2. A data cable was connected to the GARMIN 72 GPS unit and to
the computer and the GPS unit (simulator mode is fine) was turned on.
3. The DNRGarmin program was then opened. On menu at top, GPS?
was been selected, and then Set port? tab to USB
4. Next, the type data we wished to download was clicked, such
as waypoint, track or route, then Properties? and then on the tab marked
projection?.
5. Once these points have been inserted into the program, we
then connect the points to form polygons of the various items we wished to
identify in the map.
6. The map was saved on the hard drive and then used later for
representation of the various layers which could be shown on the map..
3.5 Calculation of the water requirements in each plot
3.5.1 System requirements
The water requirements of the plots which were been irrigated
was calculated by taking into consideration the type of irrigation system
concerned as well as the size of the plot and the number of sprinklers on the
plot. AUTOCAD 2004 software was used to develop the irrigation map of each plot
and the number of sprinklers calculated. Figure 3.5 shows an irrigation map
developed with AUTOCAD 2004 software and served as the ground base for
calculating the number of sprinklers on a given plot. The number of sprinklers
was then entered into the database and the total flow rate calculated
automatically by taking the product of the flow rate, the number of sprinklers
on the plot and the efficiency of the system involved. Through queries on the
database, the total flow rate required for all the plots in production could be
calculated.
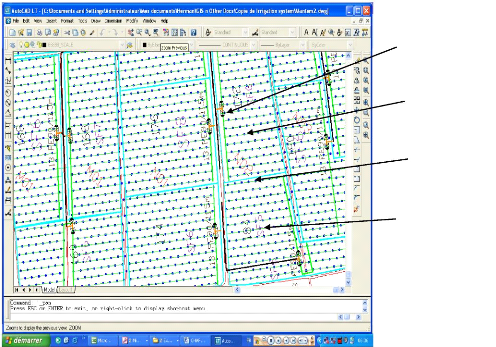
Plot valve
Sprinkler head
N02=Production plot ID
SF6=Irrigation plot ID
Figure 3.9: Irrigation map for a production plot
developed with AUTOCAD 2004
Through queries on the database, the system requirements of
the various plots were been calculated and the results of these printed up in
reports or used to create other tables which can be viewed from the forms
created. Figure 3.10 shows the environment for the creation of such queries
using Microsoft Access 2003 software.
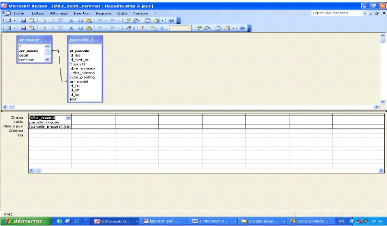
Figure 3.10: Query created in MS access to obtain the
water requirements of the system The SQL query created to obtain these
values is as follows:
UPDATE arroseur INNER JOIN parcelle_irriguée ON
arroseur.arr_model = parcelle_irriguée.arr_model SET
parcelle_irriguée.Débit_Nominal = [parcelle_irriguée].
[nbre_arroseur]*[arroseur]. [débit]
3.5.2 Crop water requirements
Crop water requirements were calculated using the water balance
equation
S(j+1)-S(j)= (Peff(j)+I(j))-(ETc(j)-D(j)) (3.1)
S(j+1)-S(j): Variation of available water content
Peff(j) : Effective rainfall
I(j) : Irrigation depth
ETc(j) : Daily crop evapotranspiration
D(j) : Drainage losses
Variation in available water content was computed in the database
by taking into consideration the water content of the previous day and that of
the following day.
Effective rainfall was computed using equations 2.4 and 2.5 by
entering these equations into an excel spreadsheet together with other
meteorological data for the different weather station involved. Rainfall after
the soil has attained its field capacity and those less than 5 mm/day are being
considered non significant to the water balance. These were considered as being
lost as runoff or by drainage.
ETc(j) was calculated as a product of the crop coefficient
Kc and the reference evapotranspiration ETp.
ETp values are being entered in the climatic data table in the
database as obtained from the records from the various meteorological stations.
Corresponding Kc values for the various plots were been used in the database
for the different number of days after planting in the plots. The Kc
values used in this work are those proposed by Allen et al., 1998.
Thus, for each plot ETc is given by equation 2.7.
Irrigation depth was calculated with respect to the system in
place; for microjet sprinkler systems, a factor of 0.9 is used while for
undertree systems, a factor of 0.85 is applied and 0.8 for big gun system to
take into consideration the efficiency of each system. Thus, the irrigation
depth is the product of the quantity of water applied and the correction
factor.
On each given date, depending on the events of the previous
day (rainfall and evapotranspiration) the quantity of water to be applied was
computed for each plot.
Using 20 year climatic data, a probability test for the risk
of non satisfaction of crop water requirements was calculated by taking the
mean of these requirements within this period for each month. Appendix I shows
the procedure used in the calculation of the water requirement as entered in MS
excel.
3.6 Evaluation of the Functioning of the Network
One of the five irrigation networks was considered in this
study for analysis. Analysis of the network was done by dividing the network
into various branches and taking into consideration the number of hydrants
which are opened upstream during irrigation. Hydraulic calculations were done
based on the formulas proposed by Zoungrana (2002) while heads losses were
calculated using the Hazem-William equation. Microsoft Excel software was used
for the hydraulic analysis.
3.6.1 Calculation of flow rates
If (en m3/h) is the nominal flow rate of one big gun,
n the number of opened big guns upstream and (m3/h) the flow rate
from the extremity of the downstream branch,
the flow rate entering the upstream branch, (m3/h) is
given by the formula:
(3.1)
The flow rate leaving the downstream branch is calculated by
respecting the loop rule of networks which is derived from the principle of
conservation of matter as:
? Flow entering = flow leaving (3.2)
Given that the network functions both in the transport and
distribution of water, the hydrants have almost equal flow rates. The flow rate
which results in the same head loss
4 ? Q
? otherwise known as the fictitious or simulated flow
rate,
? ?
? 10 ? 3600
D ? (m3/h), was calculated by the
formula presented by Zoungrana (2002):
(3.3)
3.6.2 Calculation of flow velocity and head losses.
Given that the flow rate in the conduits follows steady state
conditions for the same pipe diameters (i.e. the velocity in the fluid is the
same in both magnitude and direction at a given instant and in at every point
in the fluid), the flow velocity was calculated by applying the formula:
(3.4)
Where:
= Flow velocity (m/s)
= Flow rate considered (m3/h)
= internal diameter of the conduit (mm)
Also the velocity of flow in water supply conduits is always less
than or equal to 1.5m/s so knowing the diameter of the conduits, the velocity
of flow could be generated.
, but
Head losses were being calculated using the Hazem-William
equation
(3.5)
Where,
Linear head losses (m)
L= length of given branch (m)
Q= fictitious flow rate (lps)
C=150 for plastic pipes and 120 for steel pipes D= internal
diameter of pipe (mm)
Singular head losses due to T`s, bends, valves and elbows which
are difficult to calculate are estimated to be 10%
(3.6)
Total Head Losses, J, in a given branch is the sum of the linear
and singular head
losses
(3.7)
The ratio of this head loss on the length of a given branch gives
us the unit head losses given by:
(3.8)
Where,
j = unit head loss (m/m)
L = length of branch (m)
J = head loss in a given branch (m)
3.6.3 Determination of available and required
pressures
At the level of each hydrant, the available pressures and the
required pressures were calculated as follows:
(3.9)
Where,
available pressure upstream (m)
available pressure downstream (m)
upstream piezometric elevation (m)
downstream piezometric elevation (m) = head loss in a given
branch (m)
The required pressure downstream equals zero, if the number of
big guns at the
??
downstream hydrant equals zero or equal to the required pressure
upstream if the number of big guns at the downstream hydrant is different from
zero.
The pressures available upstream used in our calculations are
those of the technical slip of the principal conduits of PHP, version 2 of
16/02/01.
These calculations were done taking into consideration the
canon or the plot with the worst case (i.e. the furthest big gun on relatively
flat ground or the most elevated or both). The other systems such as the
microjet and undertree systems were been converted into the equivalent number
of big guns. This is because this system sets the ground base for the
conversion to other systems.
For undertree irrigation systems, the pressure required at the
entry of a lateral is given by the formula proposed by Azenkot (1999),
considering that the terrain is relatively flat.
hu = hs + 3/4F + r (3.10)
Where,
hu = pressure required at the entry of the
lateral (m) hs = nominal pressure of sprinkler (m)
F = correction factor
= linear head loss in the lateral (m)
r = height above the ground of the sprinkler
(m)
The pressure required at the center of the primary conduit is
calculated in the same way as that of the laterals and is given by:
hr = hu +3/4(Fr ) (3.11)
Where,
hr = required pressure at the centre of the lateral (m) Fr =
correction coefficient of the lateral
= head losses in the lateral (m)
? P avn ? Z av
The pressure available at the entry of the secondary conduit or
hydrant is given by equation 3.2:
= hr + + Zp - z (3.12)
Where,
= pressure required at the hydrant (m)
= head losses in the secondary conduit (m)
Zp = geometric elevation of the hydrant
z = geometric elevation of the plot with the worst case (m)
For irrigation plots totally covered by big guns, the required
pressure is calculated as above, the only exception being that the correction
factor is considered to be unity (1).
3.6.4 Calculation of piezometric elevations
The piezometric elevations are calculated based on the following
formula proposed by Zoungrana, 2002
. piezometric elevation required downstream
(3.13)
Where,
piezometric elevation required downstream (m)
required pressure downstream (m) downstream elevation (m)
. piezometric elevation required upstream
(3.14)
Where,
required piezometric elevation upstream (m)
required pressure downstream (m) upstream elevation (m)
. available piezometric elevation upstream
(3.15)
Where,
available piezometric elevation upstream (m)
required piezometric elevation downstream (m)
= head losses in the given branch (m) . piezometric elevation
retained upstream
max ( , ) (3.16)
Where,
piezometric elevation retained (m)
3.7 Spatial Representation of some Aspects on the
Irrigation System
Spatial representation of some aspects on the irrigation
system was done under MapInfo and AutoCAD software. Multiple queries on the MS
access data base was carried out to be able to obtain the data that needed to
be represented spatially. An ODBC connection between the database and the
MapInfo software was created to be able to represent these aspects of the
irrigation system. Queries led to the creation of tables in database formats
(dbf) which helped to link them to the land use map of the plantation. This
is because tables are linked by the plot ID which is a common
denominator to both tables. For example, to represent spatially the plots which
have the microjet irrigation system, a query was created on the plot table
which has one of its columns being the plot ID same as that for the map
table.
CHAPTER IV RESULTS AND DISCUSSIONS
4.1 Database for the Irrigation System
The process of development of a database for the system led to
the realization of a model for the data concerning the irrigation system.
4.1.1 Physical model
The physical model of data shows how the various tables of the
database have been linked based on the relationships that exist between the
various entities in the tables (Figure 4.1). It could be seen from this model
that a zone for example, will comprise several plantations, a plantation will
comprise several sectors etc. These relationships thus developed govern the
mode of querying data for the irrigation system. Based on the information that
is required at any point in time, the irrigation manager could query tables
that are linked to the information that is required for the system.
The relationship between the different tables is presented as
it was lastly defined and thus, is not standard. The fields of the different
tables are equally not final and modifications could be envisaged after a trial
period of the GIS database. This method of organizing data will greatly improve
management of the irrigation system as the irrigation manager could simulate
any field situation and obtain the information that is required.
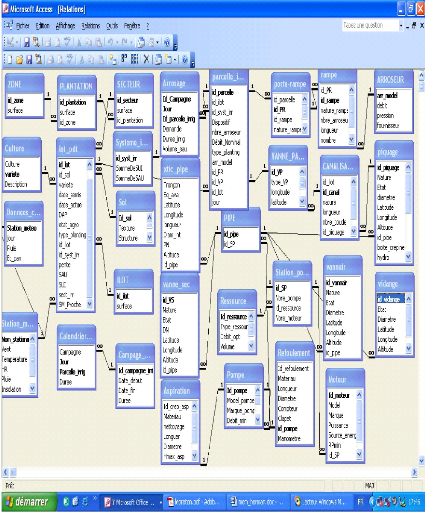
Figure 4.1: Presentation of the physical model of data as
developed in MS Access
4.1.2 Creation of forms
Ten forms for the irrigation system were created and the layout
of one of these forms is presented in Figure 4.3.
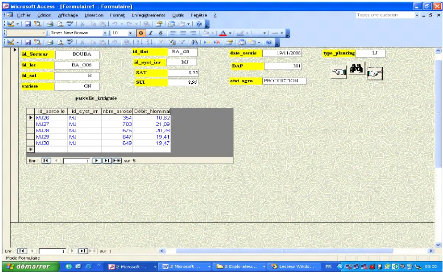
Figure 4.2: Form for data entry and retrieval for the
production plot
Forms bring a number of advantages to the data entry table:
· Because the form shows only one record at a time, the
irrigation manager can almost always see all the table fields at once,
contrary, to datasheets were it is only possible to see only four or five
columns at a time.
· Controls such as drop-down lists reduce the possibility
of data entry errors by giving the users a limited set of choices for a
field.
· Access will give irrigation managers a number of
customization options. These features let them create Access forms that look
exactly like paper forms, and they can add graphics and other objects to make
the forms more interesting.
· The irrigation manager will not be distracted by other
data in the table, and thus give full attention to the task at hand.
Specifically to the irrigation database, the forms give users
the possibility to enter and retrieve information without having to open the
tables involved. One could for example want to know the plots that are
irrigated in a particular production plot, to replace a plot that is now under
fallow, to enter a new irrigation plot etc. This will be easily done with the
use of forms. Some of the forms created included that of the irrigation plots,
production plots, the age of the plantation and a total of 10 forms were
created to enhance data entry and retrieval.
4.2 Thematic layers for the GIS
Tables 4.1, 4.2 and 4.3 show the various data that have been
entered into the database to constitute the thematic layers for the GIS. The
various layers have different uses in the database such as the geographical
location of the various soil types, equipments on the irrigation system,
creation of water requirement maps, digital elevation maps, and determination
of ETc etc.
Table 4.1: Thematic layers for simulation of the
functioning of the network
Thematic layer of GIS
|
Mode of representation
|
Principal objectives
|
Data source
|
Hydrants
|
Vector (polyline)
|
Visualize in a georeferenced space the various hydrants
|
Irrigation Service
|
Irrigation
|
|
Visualize in a georeferenced
|
|
Network
|
Vector (polyline)
|
space the various equipments of the water supply system
|
Irrigation Service
|
|
|
Visualize access for
|
|
Road network
|
Vector
|
maintenance works on the
|
DST
|
|
(polyline))
|
Irrigation system
|
|
Contour lines
|
Vector
|
Create a DEM to determine the altitude at each point in
|
|
|
(polyline)
|
the plantation
|
DST
|
Equipments on
|
Vector
|
Visualize in a georeferenced
|
Irrigation
|
Irrigation System
|
(polyline)
|
space all equipments on the primary, secondary
and tertiary network
|
Service
|
|
shp=shape file, dbf=database format, DEM=Digital elevation
model, GN=Grand Nain cultivar, W=William cultivar
Table 4.2: Thematic layers needed for water balance
calculations
Thematic layer of the GIS
|
Mode of representation
|
Principal objective(s)
|
Data source
|
Zonal boundaries of
|
|
|
|
PHP Sud
|
Vector (polygon)
|
Create a DEM
|
.shp File
|
|
|
|
Associated to a
|
|
|
Geographically locate all objects present in this limit
|
.dbf File
|
Boundaries php1-2-3-4-5
|
Vector (polygon)
|
Create a DEM
|
.shp File
|
|
|
|
Associated to a
|
|
|
|
.dbf File
|
Sector boundaries
|
Vector (polygon)
|
Create a DEM
|
.shp File
|
|
|
|
Associated to a
|
|
|
|
.dbf File
|
Production plot boundaries
|
Vector (polygon)
|
Create a DEM
|
.shp File
|
|
|
|
Associated to a
|
|
|
|
.dbf File
|
Boundary of spatial occupation, GN cultivar
|
Vector (polygon)
|
Create a DEM
|
.shp File
|
|
|
|
Associated to a
|
|
|
|
.dbf File
|
Boundary of spatial occupation, W cultivar
|
Vector (polygon))
|
Create a DEM
|
.shp File
|
|
|
|
Associated to a
|
|
|
|
.dbf File
|
Soil types
|
Vector (polygon)
|
Create a DEM et
|
Soil map from the
|
|
|
Visualise in a
|
Direction
|
|
|
Georeferenced space the
|
Agrononiques? et de la Recherche
|
|
|
Various soil types in the plantation
|
(DAR)
|
Meteorological
|
|
|
|
Stations
|
Vector (polygon)
|
Visualise in a
|
|
|
|
Georeferenced space the various stations
|
DAR
|
|
|
Create ETP, climatic data, and water requirement maps
|
|
Rainguages
|
Vector (polygon)
|
Visualise in a
|
|
|
|
Georeferenced space the location of the rainguages
|
DAR
|
Crop developmental
|
|
Create a DEM of the production plots
|
Direction de
|
stages
|
Vector (polygon
|
concerned
|
Production (DP)
|
|
Table 4.3: Thematic layers for non-descriptive
data
Non Spatial Principal Data
data Characteristics Manipulation Simulation
objective(s) source
Crop Variety
Developmental stage Modelling
Crop coefficient Crop water Irrigation Topological DP
Height above the ground Requirements scheduling Description
Max rooting depth Statistical analysis
Min rooting depth Simulation Depletion level
Yield factor
Planting date
Soil Field capacity
Wilting point Determination of
TAWC the soil type
RAW Available Water Irrigation Calculate soil DAR
Infiltration rate Content scheduling moisture deficit
Calculate soil
% Clay moisture
% Sand Content
% Silt
Maximum rooting depth
Climate Temperature
Rainfall ETp Irrigation
Relative humidity ETc scheduling
|
Calculate crop
water
requirements and
water DAR
|
|
Wind speed loss by the plants
Sunshine hours
Effective Precipitation
Production Surface area Calculate quantity
Plot Agronomic state of water consumed
Crop variety Quantity of Irrigation Modeling DP
Planting date Water required scheduling
Spatial arrangement on the plot
Soil type
|
Water-plant- atmosphere Determination of the terms
of the water balance equation
|
|
Water deficit Calculate irrigation
Slope Efficiency Irrigation system
4.3 Water requirements in each plot 4.3.1 System
requirements
The system water requirements vary with respect to the system
in place. From the database, the quantity of water that was needed by each plot
was obtained. The number of sprinkler heads which function simultaneously and
the efficiency of the system concerned were considered in these calculations.
Figure 4.3 shows a report of a query to calculate the system requirement using
MS Access 2003.
The various systems found in the PHP group and their
characteristics are: Big gun (canon) system
Rain bird big guns: 60 m3/h at 5 bars
Rainfall depth: 10 mm/h
Microjet system
Rondo type: 300 l/h at 1.5 bars
Rainfall depth: 3 mm/h
Undertree system
Rain bird type: 620 l/h at 3.0 bars
Rainfall depth: 4.78 mm/h
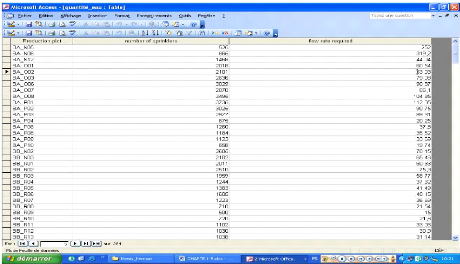
Figure 4.3: System water requirement as calculated in MS
access
4.3.2 Crop water requirements
Climatic data for a period of 20 years (1989-2008) from
meteorological stations in the plantation were used in this study. These
stations provide rainfall and pan evaporation data.
The average annual rainfall varies from 2400 mm to 3200 mm and
distributed as thus in the plantation, with Mantem being the highest in
elevation and Bonanadam the lowest in altitude:
Mantem : 3200 mm/yr
Loum : 3200 mm/yr
PHP-haut : 2800 mm/yr
Dia-Dia, Bonandam : 2600 mm/yr
Sir, Mpoula : 2500 mm/yr
Four : 2400 mm/yr
There seems to exist a relationship between altitude and
rainfall and enables us to distinguish the high altitude plantations (Mantem
and Loum), low altitude plantations (DiaDia, Bonandam, Mpoula, Sir and Four)
and intermediate altitude plantations (PHP-haut). The evapotranspiration was
calculated in the database by applying a pan coefficient, Kp of 1.1 as
indicated by Allen et al. (1998) and the crop coefficient as given by
the database.
The RAW was found to vary by a factor of 10 with respect to
the type of soil and by a factor of 4 with respect to root depth. The RAW was
considered to be 10 mm for every 10 cm of root depth.
A probability study for the risk of non satisfaction of the
crop water requirements was done on the 20 years climatic data available. All
the rainfall data was assumed to be effective because of the following
reasons:
· Rainfall in the dry season are the most susceptible to
modify the terms of the water balance equation. We thus considered that during
this period due to the physical properties of the soil (high water retention,
high hydraulic conductivity), the quantity of water loss as run-off and
drainage is negligible.
· Rainfall data obtain were already cumulated for each
month and it was therefore difficult to distinguish rainfall that are less than
5 mm/day as proposed by Smith et al. (1998).
· Most of the soils of the group are andosols and
hence have a high capillarity. This upward movement of water is thus considered
to compensate for the non effective rainfall.
Table 4.4 gives the probability of satisfaction of irrigation
requirements for a total of 20 years so as to better schedule irrigation with
the use of the database knowing the risks that could arise I these water
requirements are not fully satisfied. The table shows a summary of the average
monthly water requirements for banana in the area.
Table 4.4: Probability of satisfaction of crop water
requirements (requirements in mm)
|
Jan
|
Feb
|
Mar
|
Apr
|
May
|
Jun
|
Jul
|
Aug
|
Sep
|
Oct
|
Nov
|
Dec
|
1 yr/2
|
>102
|
>69
|
0
|
0
|
0
|
0
|
0
|
0
|
0
|
0
|
>31
|
>98
|
1 yr/5
|
>137
|
>127
|
>50
|
0
|
0
|
0
|
0
|
0
|
0
|
0
|
>82
|
>127
|
1 yr/10
|
>144
|
>139
|
>65
|
=0
|
=0
|
=0
|
0
|
0
|
0
|
0
|
>123
|
>139
|
1 yr/20
|
172
|
138
|
124
|
64
|
34
|
36
|
0
|
0
|
0
|
9
|
133
|
149
|
|
If we consider a probability of satisfaction of crop water
requirements 1 yr out of 5, then we need to apply a total of
137 mm of water per month, an average of 32 mm/week.
If this risk of satisfaction is considered to be 1
yr/10, the quantity of water to be brought in through irrigation is
144 mm per month, giving an average of 33.5 mm/week.
Taking a risk factor of 1:20, that is, satisfying the crop
water needs 1 out of 20 years, the water required by the crops will be 172 mm
per month giving an average requirement of 40.2 mm/week for an
average root depth of 50 cm.
With respect to the irrigation systems this water requirement
could further be adjusted by applying the efficiency of the system,
Keff. Table 4.5 thus shows the weekly dose of water to be applied
taking into consideration the various satisfaction probabilities and the
irrigation system concerned.
Table 4.5: Irrigation dose (mm) for two irrigation
systems
Probabilty of non satisfaction Undertree
Microjet
of irrigation water Keff =0.8 Keff =0.9
requirements
1 year/2
|
30
|
26
|
1 year/5
|
40
|
35
|
1 year/10
|
42
|
37
|
0 risk
|
50
|
45
|
|
This water requirement is been fractioned and applied three
times in a week for soils with light textures as shown in the irrigation
calendar in Appendix II. Thus for an application of 40 mm a week, the
application could be 13.3 mm in 3 days.
When this water requirement is calculated, depending on the
events of the previous day, the value is adjusted in the database. If for
example, we simulate a situation where the effective rainfall is say 10 mm and
that ETc is 4 mm then for a 21.3 mm crop demand, we would apply only
7.7 mm after a reduction of Peff and ETc. This shows that
7.7 mm of water could be saved. This could lead to reduction in the pumping
time and cost of operation and increase in marginal profits.
4.4 Simulation of the Functioning of the Network
Analysis of the network shows that the total length of the
networks is 7 056 m and that the total amount of water that could be
transported in the whole network is 5 313 m3/h. With a simulated
manometric height of 240 m, the service pressure is 24 bars, while the total
head losses in the network is 19.7 m. This gives a loss in pressure of
approximately 2 bars. The flow velocity in the various branches vary from 0.8
m/s - 1.1 m/s and are in accordance with the norm ( ) for flow in closed
conduits described by Zoungrana, (2002).
The different parameters calculated and the hydraulic
characteristics of the network are presented in Appendix III. The flow
velocities, the roughness coefficient, the deviation in pressure at the level
of the hydrants, the cumulated head losses and the total manometric height are
the principal characteristics emphasized on.
The manometric height of the pumping station is obtained as
the difference between the retained piezometric elevations upstream and the
geometric elevation of the pumping station. Where ever the manometric height
considered is less than that which is calculated, the network is said to be mal
functional. Where total head losses in the pipe are less than 10% of the
service pressure at the pumping station (HMT), and where there exist
insufficient pressures in a hydrant, a suppressor will be needed in order to
increase the available pressure at the hydrant. Where head losses are greater
than 10% of service pressure at the pumping station and that the pressures at
the hydrants are sufficient or not, these pressures need to be reduced.
4.5 Spatial Representation of some Queries on the
Irrigation System
The database created helps the irrigation manager to inquire
information related to irrigation project in GIS environment. Either Permanent
data or yearly changing data can be inquired in the database. Some examples of
created queries are as follows:
- Parcel irrigated during a given production year
- Crop pattern under selected channel
- Geographical position of plot valves
- Type of irrigation systems in various plots
- Crop pattern under selected parcel
4.5.1 Spatial representation of crop coefficients
The sensibility of crops to water stress is represented
spatially as show in Figure 4.4 by considering the crop coefficients for the
various production plots and the soil types involved. This helps managers in
the localization of plots with critical conditions and hence enhances decision
making. Thus, in the case of water shortage and only few plots could be
irrigated, the plots with a critical response to stress will be satisfied
first. This done through a query of the database and the result presented in
the GIS. Figure 4.4 therefore illustrates that in order to take a management
decision for irrigation, the plots shaded in green on the map have to be
irrigated before those in orange. These plots either have plants with a higher
vulnerability to water stress or are found on plots whose soils are of light
texture and thus need frequent irrigations.
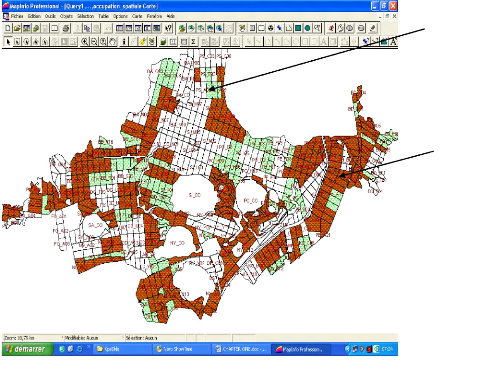
Plots with a higher sensibility to water
Lower sensibility
Figure 4.4: Sensibility of various plots to water stress
with respect to Kc
4.5.2 Spatial representation of some plot valves
For interventions on the plots, prompt actions could be taken
if the exact valves that need to be opened or closed are being represented
graphically as shown. When water becomes a limiting factor, particular plots
which to be privileged could thus be quickly identified and satisfied. This is
obtained through queries on the database and the results represented spatially
on map as shown in Figure 4.5. In case of a repair intervention on a given
plot, the exact valve to be closed is easily recognized geographically from the
map. Another utility of such a map could be in the regulation of pressure on a
given plot during periods of water shortages, by closing the valves which have
plants which are less sensitive to water stress and opening those of plots with
a high sensitivity to water stress.
Figure 4.5: Plot valves for two irrigation
plots
4.5.3 Theissen polygon for rainfall heights on the
plantation
Rainfall heights entered in the database from the different
meteorological stations are being interpolated to get the rainfall in
plantations with no rain gauges using the MapInfo GIS software. The
distribution of rainfall within the different plots in the plantation could be
obtained spatially and integrated into maps to get the rainfall depths for
other portions of the plantation with no rain gauges. Figure 4.5 shows the
distribution of rainfall depths in the plantation after a rainfall in the
plantation. The irrigation manager uses this information to adjust the water
requirements of each plot in the water balance equation by knowing the amount
of rainfall that is received each plot. This is to minimize energy used in
pumping of water and hence to maximize profits from the sale of bananas.

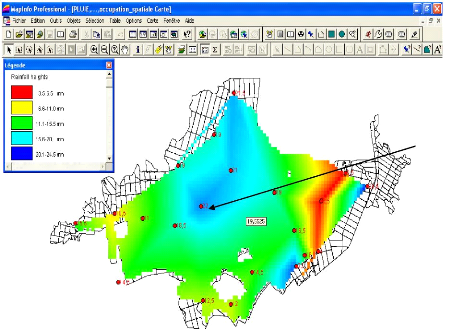
Position of rain guage
Figure 4.6: Repartition of rainfall depths in the
plantation
CHAPTER V: CONCLUSIONS AND RECOMMENDATIONS 5.1
Conclusions
Based on the methodology used and the analysis of results:
· A total of 17 tables for the various aspects
pertaining to irrigation management have been developed for the database. The
main aspects such as the crop coefficients of the various production plots, the
irrigation systems involved etc. These tables developed help in enhancing data
entry and retrieval through the use of forms and queries.
· Thematic layers for the GIS such as the soil types,
the boundary/limit of the various plots have been determined by the use of a
map of the plantation developed with the use of a GPS. This land use map
constituted the main thematic layer for the GIS.
· The crop water requirements were calculated to be 40
mm for an average rooting depth of 50 cm for the irrigation of banana in the
area. The probability of satisfaction reveals that, this depth of water will
satisfy crop water requirements 1 year out of 20. The water requirement will be
adjusted in the database depending on the ETc and Peff of
the previous day or week.
· Analysis of the existing network revealed that, the
system in place is functioning properly considering the pressures and flow rate
required. The flow velocities (0.8=V= 1.1 m/s) in the different branches of the
network show that they are satisfactory. This shows that if there exist
deficiencies in the system this could be only due to scheduling options as
earlier stated by Sisodia, (1992).
· Maps have been developed for plot valves, position of
rain gauges in the plantation, the type of irrigation system involved, the
sensibility of the various plots to water stress and other aspects related to
the irrigation system and which are necessary for the calculation of the water
requirements have been represented spatially to help irrigation managers in the
aspects of irrigation scheduling. Maps for any event on the plantation could
thus be printed out and given to the technicians for execution of particular
tasks on the irrigation system.
The irrigation network has undergone some form of evaluation
and monitoring. For instance, monitoring of the flow rates in the main
irrigation canals has been carried out. If
this type of information is available in a GIS format,
evaluation and monitoring can be made easier, timely and cost-effective.
5.2 Recommendations
5.2.1 Improvement of the system
· Organization of data for the system should be a main
priority for the Group. This will help to have more realistic values for use in
the GIS.
· Rain gauges should be redistributed in the plantation
in order to get a more coherent repartition for rainfall data which forms one
of the basic entities of the water balance equation.
· An internal network between computers should be
created with the various sources (DST, DAR, DP) such that, information should
be readily accessible. This will decrease the time needed for obtaining
information for the installation of a particular irrigation system on any
portion of the plantation and the monitoring of its water requirements
· Tensiometers need to be installed in the production plots
to compute the adequacy between the water supplied to the banana plants and
that which is consumed.
5.2.2 Further research
· A user interface between the MS access database and the
Mapinfo GIS software needs to be created to give a more user friendly and
convivial work environment.
· The database needs to be equally linked to decision
models such as hydraulic and agro-economic models to help managers in the
understanding of which option to use when confronted with several decision
options.
REFERENCES
Adam, H., Beaudequin, D., 1997. Matériels et
Systèmes d`Irrigation et Drainage. In Rôle et Objet de
ISO/T3/SC18. Proceedings of the ICID-ISO Workshop on the Standardization of
Irrigation Equipment, 11 September 1995. FAO Water Reports N° 8, FAO,
Rome, Italy, 76 pp.
Allen, R.G., Pereira, L.S., Raes, D., Smith, M., 1998. Crop
Evapotranspiration: Guidelines for Computing Crop Water Requirements. FAO
Irrigation and Drainage Report, Rome, 300 pp.
Alvanker, R., Mousavizadeh, M. H., Nazari, M., 2000.
Application of GIS in Water Resources Studies. Fourth Conference of Iranian
Commission on Large Dams. January 5-10, 2000, Tehran, Iran. 48 pp.
Amor, V. M., DAS GUPSTAL, A., LOOF, R., 2002. Application of
GIS and Crop Growth Models in Estimating Water Productivity. Journal of
Agricultural Water Management 54, pp. 205 -225.
Anonymous, 1998. Estimation des Chiffres Mondiaux de Production
et Commercialisation de Banane. FRUITROP 51, pp. 9-10.
Anonymous, 2003. Dossier Filière Banane. In Cameroon
Tribune, N° 7841/4130, 31pp.
Aquastat, 2009. FAO Information System on Water and Agriculture,
FAO, Rome. Obtainable from
www.aquastat.org
Atangana, J. B., 2003. Présentation et Etude
Comparée de quelques Dispositifs de Plantation du Bananier (Musa
acuminata Colla) : Cas des Plantations Industrielles de la SBM-Loum.
Unpublished « Ingénieur Agronome » thesis, Department of Crop
Science, University of Dschang, Dschang, Cameroon. 58 pp.
Azenkot, P. M., 1999. Irrigation, Guide Pratique. 2e Edition.
CEMAGREF, Antony; edition France Agricole, Paris. 245 pp
Beernaert, F., Bitondo, D., 1993. Land Evaluation Manual.
Department of soil Sciences, University of Dschang, Dschang, Cameroon, 212
pp.
Boa, A. P., 2005. Optimisation des Pertes de Charges dans le
Réseau d`Irrigation des Plantations du Group PHP-SBM de Njombé.
Unpublished « Ingénieur Agronome » thesis, Department of
Agric. Engineering, University of Dschang, Dschang, Cameroon. 101 pp.
Borg, A. H., Grimes, D. W., 1986. Depth Development of Roots
with Time: An Empirical description. Transactions of the American Society of
Agricultural Engineers 29 (1): pp. 194-197.
Chang, K., 2007. Introduction to Geographic Information System,
4th Edition. McGraw Hill, London. 231 pp.
Codd, E. F., 1970. A Relational Model of Data for Large Shared
Data Banks. Communications of the ACM 13 (6): pp. 377-387.
Collazzi, P. D., Sscheider, A. D., Howell, T. A., Evett, S.
R., 2003. Comparison of SDI, LEPA, and Spray Efficiency for Grain Sorghum. ASAE
Annual International Meeting. July 27-30, 2003, Las Vegas, Nevada. American
Society of Agricultural Engineers. Central Plains Irrigation Association,
Colby, Kansas. pp. 54-69.
Cremona, V.M., Witich, K.P., Sourell, H., 2000. The use of the
crop water stress index in humid climates. Zeitschrift für
Bewässerungswirtschaft 34. Zurich, Germany. pp. 175-192.
Daneshkar, A., P., Shokoohi, A., Hassandvand, A. M.,
Saghafian. B., 2000. Application of GIS and Geostatistics in Groundwater
modelling. GIS Conference in Iran, February 24-29, 2000, Tehran, Iran. 43
pp.
Dayyani, S., Mouhammadi, K., Mousavizadeh, M. H., 2003.
Irrigation and Drainage System Management using GIS. Presentation at the
CSAE/SCGR meeting, July 6-9, 2003. Montréal, Québec. 37 pp.
Defo P. M., Marie P., 1998. Proposition des techniques de
préparation des sols pour planting, Direction des Services Techniques
PHP, Njombe, Cameroun. 10pp.
Delvaux, B., 1995. Soils. In Gowen, S. R. (ed.), Bananas and
Plantains. Chapman and Hall, London. pp. 230-257.
Doorenbos, J., Kasam, A. H., Bentvelsen, C. L. M., Branscheid,
V., Plusje, J. M. G. A., Smith, M., Uittenbogaard, G.O., Van Der Waal, H. K.,
1986. Yield Response to Water. FAO irrigation and drainage paper 33, Rome. 234
pp.
Encarta, 2008. Microsoft Encarta and student program manager one
Microsoft way. Microsoft Corporation. Redmond, USA.
ESRI, 1996. ArcView GIS: The Geographic Information System for
everyone. Environmental Systems Research Institute, ESRI, Inc., Redlands, USA.
67 pp.
Evans, R., Sneed, R. E., Cassel, D. K., 1996. Irrigation
Scheduling to Improve Water and Energy Use Efficiencies. North Carolina
Cooperative Extension Service, AG 452-4. 134 pp.
Ewane, E. H., 2008. Evaluation of the Undertree Irrigation
System of the CDC Ekona Banana Plantation (EBP) Mussaka. Unpublished
Ingénieur Agronome? thesis, Department of Agric. Engineering, University
of Dschang, Dschang, Cameroon. 67 pp.
Fonsah, E. G., and Chidebelu A. S. 1995. Economics of Banana
Production and Marketing in the Tropics: A Case Study of Cameroon. Minerva
Press London. 230 pp.
Fonteh, F. M., Assoumou, E. E., 1996. Irrigation and Drainage:
Distance Education Course Notes, DE 011GR. University of Dschang, Dschang,
Cameroon. 172 pp.
Google Earth, 2009. Google earth Version 5.0.11337.168 (beta).
Data: US Navy, Europa Technologies, Tele Atlas, Map Link. Google Corporation.
USA.
Hanks, R. J., Ashccroft G. L., 1980. Soil Water and
Temperature Application. In Bommer. D. F. R., Sabey. B. R., Thomas. G. W.,
Vaadia Y., (Eds.). Applied Soil Physics. Springer. New York. 189 pp.
Heywood, I., Cornelius., Carver, S., 2006. An Introduction to
Geographical Information Systems, 3rd Edition. Prentice Hall,
London. pp. 195.
Hoogenboom, G., Jones, J. W., Boote, K. J., 1991. A Decision
Support System for Prediction of Crop Yield, Evapotranspiration, and Irrigation
management. In: Ritter, W. F. (Ed.) Irrigation and Drainage (Proc., 1991 Nat.
Conf,), American Society of Agricultural Engineers, New York: pp. 198-204.
Howell, T. A., 2003. Water Loss Comparisons of Sprinkler
Packages. Central Plains Irrigation Short Course and Exposition. February 4-5,
2003, Colby, Kansas. 98pp.
James, D. W., Hanks, R. J., Jurinak, J. J. 1982: Modern irrigated
soils. A Willey - Interscience Publication. John Wiley & Sons, New York.
345 pp.
Jensen, M. E., 1981. Irrigation Scheduling for Water and
Energy Conservation in the 80s: In Fereres, E. 1996, Irrigation scheduling and
its impact on the 21s century. Proceedings of the international conference of
the American Society of Agricultural Engineers. San Antonio, Texas, USA,
November 3 - 6.
Kopec, A. R., Langley, M. N., Bos, M. G., 1984. Major
Variables which Influence Effective Precipitation. ICID Bulletin 33 (2): 68 -
84. In: Rojas, R., Rolda'n, J. (eds.). Evapotranspiration mapping and
irrigation scheduling for olive trees. Evapotranspiration and irrigation
scheduling. Proceedings of the international conference of the American Society
of Agricultural Engineers. San Antonio, Texas, USA, November 3-6.
Kramer, P.J. (1983). Water Relation of Plants. Academic Press
Inc, New-York, USA. 489 pp.
Lane, I. E., 1955. Genera and Generic Relationships in Musaceae.
Mitt. Bot. Staatssman 45, Muchen, Germany, pp. 141-131.
Lassoudiere, A., 1979. Croissance et Développement du
Bananier Poyo en Cote d`Ivoire. These, Université Nationale de Cote
d`Ivoire, Abidjan, Cote d`Ivoire. 312 pp.
Lemeilleur, S., Temple, L., Kwa, M., 2003. Identification of
Banana Production Systems in Urban and Peri-urban Agriculture in
Yaoundé. The International Journal on Bananas and Plantains
(INFOMUSA), 12:1, pp. 13-16.
Marty, P., 1983. Fiches Techniques d`Agriculture Spéciale.
Le Bananier, Série 2, Ministère de la coopération, Paris,
pp. 3-15.
Menenti, M., AzzaliI, S., D`Urso, G., 1995. Management of
Irrigation Schemes in Arid Countries. In: Vidal, A. (Ed.) Use of Remote Sensing
Techniques in Irrigation and Drainage. FAO, Rome, pp. 1-98.
Merriam, J., Keller, J., 1975. Farm Irrigation Evaluation: A
Guide for Management. Utah University Press, Utah, USA. 156 pp.
Molden, D. J., Saktivadivel, R., Christopher, J., Perry, De
Fraiture, C., Kloezen, W. H., 1998. Indicators for Comparing Performance of
Irrigated Agricultural Systems. Research Report N° 20, International Water
Management Institute, Colombo, Sri Lanka. 35 pp.
Moon, D., Van Der Gulik, W., 1996. Irrigation scheduling using
GIS. In Evapotranspiration and Irrigation Scheduling. Proceedings of the
International Conference of the American Society of Agricultural Engineers. San
Antonio, Texas, USA, November 3-6. pp. 644-649.
Njila, R. C., 1999. Evaluation du Systéme d`irrigation
par aspersion à la Tiko banana plantation. MSc. thesis, Faculty of
Agronomy and Agricultural Sciences, University of Dschang. 97 pp.
Pedro, A., Cora, D., Pascal, L., Paul, P., 2003. The World Banana
Economy 1985-2002. FAO, Rome, Italy. 55 pp.
Plauborg, F., Andersen, M. N., Heidmann, T., Olesen, J. E,
1996: MARKVAND: A Decision support system for irrigation scheduling.
Evapotranspiration and irrigation scheduling. Proceedings of the international
conference of the American society of agricultural engineers. San Antonio.
Texas. USA. November 3-6. pp. 527-535.
Ray, S. S., Dadhwal V. K., 2001. Estimation of Crop
Evapotranspiration of Irrigation Command Area Using Remote Sensing and GIS.
Journal of Agricultural Water Management, 49: pp. 230-249.
Rieul, L., Delacourt, A., Revol, P., Ruelle, P., 1992.
Prévention de la Pollution des Eaux par Ruissellement et par Percolation
hors d`atteinte du système Racinaire dans le cas des Cultures
irriguées. Proposition pour le code de la bonne pratique agricole,
Annexe 2, Paragraphe 10. CORPEN Editeur, Paris. 192 pp.
Riopel, J. L., Steeves, T. A., 1964. Studies on Roots of Musa
acuminata cv. =Gros Nain`. Ann. Bot. Lond., NS, pp. 90-115.
Robinson, J. C., 1981. Water Requirements of Bananas. Agriculture
and Fisheries Department, Pretoria, South Africa. 57 pp.
Robinson, J. C., 1987. Root Growth Characteristics in Banana.
ITSC Information Bulletin, N° 183, Wallingford, UK, pp. 7-9.
Robinson, J. C., Alberts, A. J., 1987. The Influence of Under
Tree Canopy Sprinkler and Drip Irrigation Systems on Growth and Yield of
Bananas (Cultivar Williams) in the Sub Tropics. Sci. Hort. 32. pp. 49-66.
Robinson, J. C., Anderson, T., 1991. The Influence of
Temperature on Dry Matter Assimilation and Distribution in Young Banana Plants.
Newsletter of the International Group on Horticultural Physiology of
Banana, 14. pp. 36-37.
Robinson, J. C., De Villier, E. A., 2007. The Cultivation of
Bananas. ARC-Institute of Tropical and Sub Tropical Crops, Du Roi Laboratory,
Pingwe Print, Nelspruit, South Afica. 258 pp.
Rojas, R., Rolda`n, J. 1996: Evapotranspiration mapping and
irrigation scheduling for olive trees. Evapotranspiration and irrigation
scheduling. Proceedings of the international conference. American society of
agricultural engineers. San Antonio, Texas, USA, November 3-6. 278 pp.
Sarangi, A., Rao, N. H., Brownee, S. M., Singh. S. A., 2001.
Use of Geographic Information System Tool in Watershed Hydrology and Irrigation
Water Management. Retrieved 24th April 2009 from
http://www.GISdevelopment.net
.
Schwab, G. O., Fangmeier, D. D., Elloit, W. J., Frevert, R.K.,
1993. Soil and Water Conservation Engineering, 4th ed. John Wiley
& Sons, Inc. New York. 234 pp.
Sisodia, J. S., 1992. Performance Monitoring Study of the
Warabandi Irrigation Management System of in the Chambal Area. Indian
Journal of Agricultural Economics, 47:4. Calcutta, India, pp. 23-45.
Smith, M., Pereira, L.S., Berengena, J., Her, B., Goussard,
J., Ragab, J., Tollefson, L., Van, H., 1996. Irrigation Scheduling: From Theory
to Practice, FAO International Commission on Irrigation and Drainage, Rome. 384
pp.
Stover, R. H., 1979. Pseudostem Growth, Leaf Production and
Flower Initiation in the Grand Nain Banana. Trop. Agric. Res. Services
(SIATSA), La Lima, Honduras. 118 pp.
Stover, R. H., Simmonds, N. W., 1987. Bananas (3rd
Ed.). John Wiley & Sons Inc, New York, USA. 468 pp.
Summerville, W. A. T., 1939. Root Distribution of Banana.
Quensl. Agric. J., pp. 70-96.
Swennen, R., Vuylteke, D., 2001. Le Bananier. In Plantes à
Fruits. Agriculture en Afrique, pp. 611-637.
Taylor, B. B., 2005. Comprehensive Water Resource Management
through Geographic Information Systems. Proceedings of the 2005 Georgia Water
Resources Conference, held April 25-27, 2005, at The University of Georgia,
Georgia, Athens. 123 pp.
Tchiadje T. N., 1995. Optimisation de l`exploitation des
réseaux d`irrigation de la SPNP. Rapport de stage d`insertion
professionnelle. INADER, Université de Dschang, Cameroun.
Thome, P. G., 2007. Etude Comparée de Deux Dispositifs
de Système Micro Jet dans une Bananeraie Plantée en Ligne
Jumelées au Group PHP-SBM. Unpublished « Ingénieur Agronome
» thesis, Department of Agric. Engineering, University of Dschang,
Dschang, Cameroon. 78 pp.
Tiercelin, J. R., 1997. L`Irrigation des Parcs et Jardins.
Lavoisier Technique de Documentation, Paris, pp. 345-415.
Tron, G., Isberie, C., Chol, P., 2000. La Tensiométrie
pour Piloter les Irrigations: Une Gestion Raisonée de la Ressource en
Eau. Educagri editions, Dijon Cedex, France. 247 pp.
Turner, D. W., Lahav, E., 1983. The Growth of Banana Plants in
Relation to Temperature. Australian Journal of Plant Physiology, 10.
pp. 43-53.
Valmayor, R. V., 1991. Banana Classification and Commercial
Cultivars in Southeast Asia. PCARRD Information Bulletin N°
24, Los Banos, Philippines. pp. 15-20.
Van De Pol M. J., Nsoyuni L. A., Minnemeyer S., Ngoufo
R., 2005. Atlas Forestier Interactif du Cameroun. MINEF, World
Resources Institute, Global Forest Watch. Washington. 244 pp.
Van Laere, P. E., 2003. Memento de l`Irrigation. Collection
Manuel Techniques?, direction Général de la Coopération
Internationale (DGCI). Bruxelles, Belgique. 300 pp.
Yamelith, A., 1998. Environmental Impact of the Banana
Industry: A Case study of Costa Rica. 15 pp.
Zoungrana, D. 2002. Cours d`approvisionnement en eau potable.
Destiné aux étudiants de la section infrastructures, Energie et
Génie Sanitaire. Ecole Inter Etats Ingénieur de l`Equipement
Rural- EIER (Burkina Faso)- Novembre 2002. 149 pp.
APPENDICES
7
|
|
|
|
|
|
|
|
|
|
|
|
|
|
|
|
|
|
|
|
|
|
|
|
|
|
|
|
|
|
|
|
|
|
|
|
|
|
|
|
|
|
|
|
|
|
|
|
|
|
|
|
|
|
|
|
|
|
|
|
|
|
|
|
|
|
|
|
|
|
|
|
|
|
|
|
|
|
|
|
|
|
|
|
|
|
|
|
|
|
|
|
|
|
|
|
|
|
|
|
|
|
|
|
|
|
|
|
|
|
|
|
|
|
|
|
|
|
|
|
|
|
|
|
|
|
|
|
|
|
|
|
|
|
|
|
|
|
|
|
|
|
YS
|
TH
|
ETWK
|
|
|
|
|
|
|
|
|
|
|
|
|
|
|
|
|
|
|
|
|
|
mb
|
3)
|
|
|
|
|
|
|
|
|
|
|
|
|
|
|
|
|
|
|
|
|
|
|
|
|
|
|
|
|
|
|
|
|
|
of
|
|
|
|
|
|
J
|
V
|
Zav
|
am
|
nec
|
nec
|
|
|
|
|
P
|
|
|
|
(
|
|
|
(
|
(m
|
k
|
m)
|
m/s)
|
mcE)
|
mcE
|
mcE)
|
cE)
|
cE
|
cE
|
mcE
|
mcE
|
mcE
|
mcE)
|
|
|
|
|
|
|
6
|
0,01
|
04
|
0,1
|
145
|
45
|
20
|
100
|
65
|
65
|
245
|
245
|
68,8
|
149
|
|
|
|
|
|
|
16
|
0,09
|
009
|
0,4
|
145
|
45
|
100
|
90
|
45
|
45
|
235
|
245
|
688
|
8,8
|
|
|
5
|
|
|
|
2
|
0,13
|
097
|
0,5
|
145
|
46
|
90
|
15
|
35
|
351
|
261
|
261
|
688
|
8,8
|
|
|
0
|
|
|
|
25
|
0,19
|
022
|
0,6
|
146
|
48
|
0
|
95
|
46
|
47
|
263
|
263
|
67,9
|
68
|
|
|
|
|
|
|
31
|
0,27
|
003
|
0,7
|
148
|
48
|
95
|
60
|
43
|
243
|
243
|
243
|
66,9
|
1,9
|
|
|
|
|
|
|
37
|
0,38
|
845
|
0,8
|
148
|
48
|
60
|
90
|
08
|
09,8
|
208
|
2098
|
669
|
107
|
|
|
|
|
|
|
4
|
0,46
|
79
|
,9
|
148
|
67
|
90
|
20
|
38
|
39,8
|
257
|
257
|
688
|
788
|
|
|
|
|
|
|
4
|
0,46
|
005
|
,9
|
167
|
67
|
0
|
05
|
67
|
67
|
187
|
187
|
51,5
|
152
|
|
|
|
|
|
|
4
|
0,46
|
028
|
0,9
|
167
|
67
|
0
|
20
|
67
|
67
|
272
|
272
|
51,6
|
52
|
|
|
|
|
|
|
4
|
0,46
|
226
|
0,9
|
167
|
71,2
|
0
|
60
|
67
|
682
|
191,2
|
1912
|
51,6
|
152
|
|
|
|
|
|
|
4
|
0,46
|
689
|
0,9
|
171,2
|
77
|
0
|
20
|
1,
|
71,9
|
237
|
237
|
48,6
|
149
|
|
|
|
|
|
|
4
|
0,46
|
028
|
0,9
|
177
|
77
|
0
|
20
|
77
|
77
|
197
|
197
|
435
|
143
|
|
|
|
|
|
|
4
|
0,46
|
689
|
0,9
|
177
|
82
|
0
|
00
|
77
|
777
|
202
|
202
|
43,5
|
144
|
|
|
|
|
|
|
4
|
0,46
|
496
|
0,9
|
182
|
208
|
0
|
00
|
82
|
83,5
|
308
|
308
|
392
|
39
|
|
|
|
|
|
|
4
|
0,46
|
694
|
,9
|
208
|
87
|
0
|
00
|
08
|
10,7
|
287
|
3107
|
14,7
|
115
|
|
|
|
|
|
|
48
|
0,6
|
084
|
1,1
|
187
|
87
|
0
|
09
|
0,7
|
08
|
287
|
3108
|
38,4
|
138
|
|
|
|
|
|
|
54
|
0,2
|
,01
|
0,8
|
187
|
87
|
09
|
97
|
0,8
|
08
|
296
|
3108
|
38,5
|
295
|
|
|
|
|
|
|
60
|
0,25
|
236
|
,9
|
187
|
77
|
0
|
08
|
0,
|
312
|
274
|
312
|
30,2
|
130
|
|
|
|
|
|
|
66
|
0,29
|
912
|
0,9
|
177
|
75
|
08
|
20
|
0,8
|
1,7
|
283
|
3117
|
385
|
305
|
|
|
|
|
|
|
676
|
0,31
|
471
|
1
|
175
|
69
|
0
|
96
|
1,
|
22
|
189
|
3122
|
41,4
|
141
|
|
|
|
|
8
|
|
75
|
0,38
|
568
|
1,1
|
169
|
71
|
96
|
0
|
2,
|
127
|
267
|
3127
|
47,9
|
1,9
|
|
|
|
|
8
|
|
|
|
|
1
|
171
|
7
|
|
|
|
|
5
|
|
|
6
|
|
0
|
|
81
|
|
|
|
|
|
175
|
|
|
|
|
|
|
|
|
|
|
|